Translate this page into:
Emerging therapies targeting cardiovascular risk factors to prevent or delay the onset of heart failure
*Corresponding author: Olisaemeka Zikora Akunne, BPharm Department of Pharmacy, ASK Medical and Diagnostic Centre, Federal Capital Territory, Abuja, Nigeria. olisaemeka.akunne.181526@unn.edu.ng
-
Received: ,
Accepted: ,
How to cite this article: Akunne OZ, Anulugwo OE. Emerging therapies targeting cardiovascular risk factors to prevent or delay the onset of heart failure. Am J Pharmacother Pharm Sci 2024:13.
Abstract
Cardiovascular disease (CVD) poses a significant global health concern, contributing to nearly 30% of global deaths. Its prevalence is on the rise, necessitating a deeper understanding of associated risk factors including hypertension, cardiac hypertrophy, and diabetes. Addressing these risk factors is crucial in preventing or slowing the onset of heart failure (HF), a complex chronic condition with high morbidity and mortality rates. This review aims to explore innovative strategies for preventing or delaying HF, focusing on cardiovascular risk (CV) factors. Specifically, it delves into the link between hypertension, cardiac hypertrophy, diabetes, and HF emphasizing the importance of identifying new therapeutic approaches. A comprehensive examination of existing literature, clinical trials, and experimental models forms the basis of this review providing insights into the interconnected nature of cardiovascular risk factors and the efficacy of combination therapies. Evidence from diverse sources supports the adoption of a multifaceted approach to HF prevention. The review underscores the complex associations between hypertension, cardiac hypertrophy, diabetes, and HF highlighting the need for innovative therapeutic interventions. Clinical trials demonstrate promising outcomes with synergistic therapies such as angiotensin-converting enzyme (ACE) inhibitors, beta-blockers, and sodium-glucose cotransporter-2 (SGLT-2) inhibitors showcasing improved efficacy over single-agent interventions. In conclusion, adopting a multifaceted approach to HF prevention considering the interplay of various risk factors. Such an approach holds the potential for substantial benefits including simultaneous targeting of multiple pathways, individualized care, enhanced patient motivation, and reduced healthcare costs. Further research should focus on optimizing combination therapies and identifying patient population that stands to gain the most from these interventions providing a pathway towards improved cardiovascular health globally.
Keywords
Cardiovascular disease
Heart failure prevention
Hypertension
Diabetes
Emerging therapies
INTRODUCTION
Cardiovascular disease (CVD) encompasses a spectrum of pathological conditions affecting the heart, blood vessels, and the circulatory system, and stands as the leading cause of mortality and morbidity worldwide.[1] Recent statistics indicate a concerning increase in CVD prevalence, contributing to nearly 30% of global deaths and affecting both male and female populations globally.[2-4] This rising burden is driven by factors such as population aging and the presence of multiple risk factors.[5] Various risk factors, including cardiometabolic, behavioral, environmental, social, and genetic factors, significantly contribute to the development of CVD. Traditional risk factors such as smoking, alcohol consumption, hypertension, dyslipidemia, and diabetes substantially heighten the risk of CVD.[4,5] Consequently, understanding CVD prevalence and impact is crucial in addressing this global health challenge.[5]
The prevention and management of CVD can be categorized into primary and secondary prevention. Primary prevention entails the early identification and modification of “lifestyle risk factors,” while secondary prevention focuses on appropriate risk reduction to slow disease progression. Effective prevention and treatment strategies hinge on the identification of individuals at risk of CVD and the establishment of systems that facilitate proactive management.[6]
Hypertension, cardiac hypertrophy, diabetes, and heart failure (HF) are interconnected conditions with significant implications for cardiovascular (CV) health. Elevated blood pressure (BP), a primary risk factor for various CVDs, including stroke, coronary artery disease, atrial fibrillation, and peripheral vascular disease, is also a modifiable factor in HF development.[7,8] In cases of hypertension, persistent high arterial pressure triggers the enlargement of the heart muscle, known as cardiac hypertrophy, as the heart compensates for elevated arterial resistance. Hypertension substantially increases the incidence of HF, which is responsible for a significant proportion of annual deaths in the United States and entails substantial health-care expenses.[8] Furthermore, individuals with diabetes face more than double the risk of developing HF compared to those without diabetes, even after accounting for factors such as age, hypertension, hypercholesterolemia, and coronary artery disease.[9] Research indicates that diabetes-related heart disease, or diabetic cardiomyopathy (CMP), significantly contributes to diastolic dysfunction and HF in diabetic patients.
HF, a complex chronic heart condition, results in reduced cardiac pumping or filling capacity, leading to high rates of hospitalization and mortality globally.[10] Initially, the heart responds to increased load or cardiac stress by enlarging in size and mass – a compensatory process referred to as pathological cardiac hypertrophy. This enlargement aims to normalize wall stress and maintain CV function at rest. During this compensatory stage, biochemical, molecular, structural, and metabolic changes occur to preserve cardiac function. However, chronic stress or disease eventually leads to ventricular dilation, decreased contractile function, and progression to HF.[11]
Hence, the critical need for discovering novel treatments to prevent or decelerate HF onset cannot be overstated. HF represents a substantial and escalating global health issue, characterized by a high prevalence and mortality rate. Despite advancements in medical interventions, existing therapies merely mitigate disease progression, and heart transplantation remains the only available cure.[12] Animal models simulating hypertensive heart disease are pivotal in exploring potential regenerative treatments, enabling scientists to investigate fundamental mechanisms of HF and uncover fresh therapeutic targets. In addition, existing treatment options, such as conventional pharmacological agents and left ventricular assist devices (VADs), can only postpone HF progression. Consequently, there is an urgent demand for innovative therapies capable of restoring damaged hearts and enhancing the prognosis for HF patients. Identifying and implementing new therapeutic strategies for HF is of paramount importance due to its alarming rise in prevalence and the substantial burden it places on both individuals and society. This review aims to delve into the research and development of inventive and emerging therapies targeting CV risk factors, specifically focusing on hypertension and diabetes with the objective of averting or delaying the onset of HF. It intends to emphasize the significance of inventive strategies for preventing or postponing HF, ultimately contributing to the enhancement of patient outcomes in light of this escalating global health issue.
HYPERTENSION AS A RISK FACTOR
HF remains a significant public health challenge, with its prevalence expected to rise substantially by 2030 due to an aging population, with persistently high mortality rates despite advancements in treatment.[7,13] Several risk factors are thought to precede the development of HF. Among these hypertension, commonly referred to as high BP, stands out as a major risk factor and a primary precursor to the initiation and progression of HF.
Hypertension is characterized by elevated BP levels, typically defined as a systolic BP (SPB) ≥140 mmHg and/or a diastolic BP (DBP) ≥90 mmHg. It places additional strain on the heart, leading to structural and functional alterations in the heart muscle, encompassing the hypertrophy of the left ventricle, which, if left unchecked, can evolve into HF.[14] Diastolic dysfunction and HF with preserved ejection fraction (HFpEF) are the most prevalent cardiac complications associated with hypertension, but it also heightens the risk of myocardial infarction (MI) and plays a pivotal role in the intricate network of CVD, making it a significant contributor to the pathophysiological processes culminating in HF with reduced ejection fraction (HFrEF).
Understanding the link between hypertension and HF is pivotal for preventing or delaying the onset of this debilitating condition. The most widely accepted model of hypertensive HF indicates that left ventricular diastolic dysfunction is the initial detectable manifestation of heart disease.[15] Cardiac remodeling, in response to the primary pressure overload, involves an increase in cardiac mass at the expense of chamber volume, resulting from the parallel addition of sarcomeres, ultimately leading to concentric left ventricular hypertrophy. Conversely, in response to primary volume overload (such as obesity, chronic kidney disease [CKD], or anemia), cardiac remodeling entails increased cardiac mass and chamber volume caused by the serial addition of sarcomeres, resulting in eccentric left ventricular hypertrophy.[9,15] When sustained pressure overload persists, diastolic dysfunction progresses, the concentrically remodeled left ventricle decompensates, leading to hypertensive HFpEF. On the other hand, sustained volume overload results in left ventricular dilation, leading to decompensation of the eccentrically remodeled left ventricle and the development of HFrEF [Figure 1]. Combining left ventricular hypertrophy with elevated levels of biomarkers indicating subclinical myocardial injury, such as high-sensitivity cardiac troponin T and N-terminal pro–B-type natriuretic peptide (NT-proBNP), identifies patients at the highest risk of developing symptomatic HF, particularly HFrEF.[16]
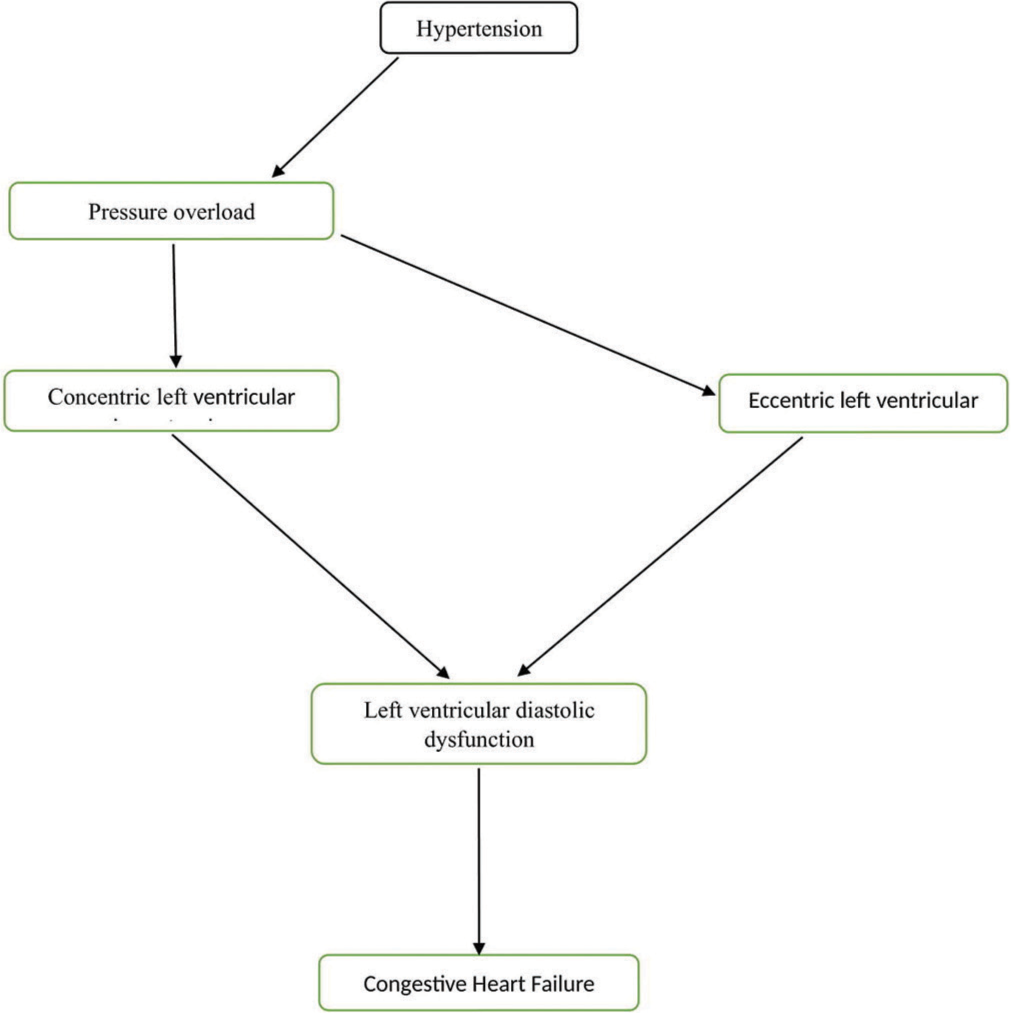
- Development of congestive heart failure from hypertension.
The final stage of hypertensive heart disease, often stemming from prolonged pressure and volume overload, manifests as dilated CMP characterized by both diastolic dysfunction and reduced ejection fraction. The connection between hypertension and HF also involves changes in the reninangiotensin-aldosterone system (RAAS), which is over activated due to left ventricular systolic wall stress and further contributes to cardiac hypertrophy. In addition, the sympathetic nervous system (SNS) plays a significant role in left ventricular hypertrophy, overt vasoconstriction, and electrolyte retention, especially sodium. These effects are induced by the compensatory activation of the SNS, which can result in structural and functional changes in the heart, including left ventricular hypertrophy and modified myocardial contractility.[17,18] Clinically, hypertensive heart disease can be categorized into four ascending degrees based on the pathophysiological and clinical impact of hypertension on the heart:
Degree I: Isolated left ventricular diastolic dysfunction with no left ventricular hypertrophy.
Degree II: Left ventricular diastolic dysfunction with concentric left ventricular hypertrophy.
Degree III: Clinical HF, characterized by dyspnea and pulmonary edema with preserved ejection fraction.
Degree IV: Eccentric left ventricular hypertrophy (dilated CMP with HF) and reduced ejection fraction.
Novel hypertension treatments
Recent research advancements in the realm of hypertension treatments have shown significant progress. These discoveries have not only deepened our understanding of hypertension’s underlying mechanisms but have also paved the way for innovative pharmacological and interventional approaches to combat this prevalent condition.[19]
To enhance hypertension treatment, both pharmaceutical companies and academic researchers have launched dedicated programs focused on developing new drugs and intervention techniques to lower BP. The goal of these initiatives is to overcome the limitations of current treatments and enhance the outcomes for individuals with hypertension. It is crucial to underscore the need for effective hypertension management, given the associated health risks, mortality rates, and societal expenses. Research in hypertension has revealed that despite the availability of effective treatments, only a third of individuals under pharmacological treatment successfully control their BP. This emphasizes the urgent need to identify new hypertension risk factors and developing preventative measures. Moreover, the high global prevalence of hypertension presents therapeutic challenges that call for the exploration of fresh treatment options.[20]
Recent research has centered on understanding the molecular mechanisms governing BP regulation. Existing antihypertensive medications do not address all prohypertensive pathways and may trigger counter-regulatory mechanisms that diminish their long-term effectiveness. As a result, there is a demand for new pharmacological strategies capable of providing additional BP reduction and offering broader CVD protection, particularly in cases involving comorbid conditions such as CKD and diabetes.[21]
Sodium-glucose cotransporter 2 (SGLT-2) inhibitors
SGLT-2 primarily functions in the kidney, where it reabsorbs filtered glucose. By inhibiting SGLT-2, the reabsorption of glucose is reduced, resulting in increased glucose clearance through urination, which lowers blood glucose levels.[21] These novel oral hypoglycemic drugs, specifically empagliflozin, canagliflozin, and dapagliflozin, represent significant breakthroughs in the realm of cardioprotective and nephroprotective anti-diabetic drugs. They have demonstrated remarkable class-effect outcomes, reducing CV and all-cause mortality, hospitalizations due to HF, and the progression of diabetic nephropathy.[22]
Although the primary focus of clinical studies involving SGLT-2 inhibitors was glucose control, secondary endpoints and safety outcomes included the effects on BP and weight. For instance, the EMPA-REG OUTCOME trial was the first to reveal a reduction in CV mortality among high-risk patients with type 2 diabetes treated with empagliflozin. This study showed a clinically significant reduction in 24-h SBP and DBP decreased by 4.2 and 1.7 mmHg, respectively, in individuals taking 25 mg of empagliflozin daily compared to a placebo after 12 weeks of treatment. A smaller, yet significant, reduction in BP was observed in those using the lower dose of empagliflozin (10 mg).[22,23] In addition, a meta-analysis of 45 placebo-controlled studies demonstrated a mean SBP reduction of −3.77 mmHg (95% confidence interval [CI] −4.65–−2.90). In six active-controlled studies, the mean change was −4.45 mmHg (95% CI −5.73–−3.18) compared to other agents. Among 16 studies, the mean change from baseline in DBP was −1.75 mmHg (95% CI −2.27–−1.23), with the six active-controlled studies suggesting a mean change of −2.01 mmHg (95% CI −2.62–−1.39) compared to other agents.[24] In summary, SGLT-2 inhibitors exert a more pronounced effect on daytime BP compared to nighttime, and their BP-lowering efficacy is comparable to that of low-dose thiazide diuretics, with a change from baseline in 24-h SBP of −3.62 mmHg (95% CI −4.29, −2.94) for SGLT-2 inhibitors and −3.46 mmHg (95% CI −6.15, −0.77) for low-dose hydrochlorothiazide (12.5 mg), while the change from baseline in 24-h DBP averaged −1.70 mmHg (95% CI −2.13, −1.26) for SGLT-2 inhibitors and −2.23 mmHg (95% CI −4.34, −0.12) for low-dose hydrochlorothiazide.[25] While the mechanisms behind SGLT-2 inhibitors’ BP-lowering effects involve natriuresis and osmotic diuresis, they are not yet fully elucidated.[26]
RAAS
The primary action of the mineralocorticoid steroid hormone aldosterone is mediated through the mineralocorticoid receptor (MR). Its core function involves maintaining the balance of sodium and potassium, which plays a pivotal role in BP regulation. Consequently, an imbalance in aldosterone levels can result in the progression of heart and kidney diseases by elevating oxidative stress, inflammatory mediators, and fibrotic factors. Extensive clinical trials have confirmed the effectiveness and safety of steroidal MR antagonists (MRAs), such as spironolactone and eplerenone, in reducing BP in individuals with resistant hypertension. However, the use of these drugs is limited due to the increased risk of developing hyperkalemia. As a result, there is a pressing need to develop innovative non-steroidal dihydropyridine compounds to mitigate the adverse effects of steroidal antagonists.[27]
Non-steroidal MRAs demonstrate a high degree of selectivity and enhanced affinity for MR, without interfering with progesterone and androgen receptors. They exhibit greater potency and a longer half-life compared to eplerenone and without the anti-androgenic effects observed with spironolactone.[22] Among these non-steroidal MRAs, finerenone stands out as the most advanced candidate with a comprehensive global clinical development program. Finerenone, a dihydronaphthyridine-based compound, exhibits a remarkable selectivity for MR over other steroid hormone receptors with a high binding affinity (IC50: 18 nM).[28] Its distinct binding mode to MR-ligand-binding domain (LBD) as a “bulky” antagonist induces a unique MR-LBD conformational change, including the protrusion of Helix 12. This binding leads to an unstable receptor-ligand complex and the prevention of transcriptional coregulator recruitment, which, in turn, triggers a ligand-specific antagonistic effect on target genes. These unique characteristics potentially explain the differential clinical responses observed with finerenone.[27,28]
Clinical trials involving finerenone have been conducted in patients with CKD, diabetes, and HFHF. Clinical trials involving finerenone have been conducted in patients with CKD, diabetes, and HFHF. Notably, in the Phase II ARTS-HF trial, among patients with HFrEF and coexisting type 2 diabetes mellitus (T2DM) or CKD, treatment with finerenone demonstrated a similar reduction in NT-proBNP compared to eplerenone. Importantly, a significant reduction in the composite of all-cause mortality, CV hospitalization, or emergency HF presentation was observed in patients receiving a dosage of 10–20 mg of finerenone compared to eplerenone. This outcome was particularly remarkable, although the trial was not specifically designed or powered to assess this endpoint.[29] Finerenone also exhibited superior results in randomized clinical trials for individuals with worsening chronic cardiac and renal conditions compared to eplerenone, reducing cardiac hypertrophy and proteinuria significantly. In addition, Finerenone demonstrated slight impacts on BP, showing average SBP alterations of −3.0 mm Hg at month 1 and −2.1 mm Hg at month 12 compared to −0.1 mm Hg at month 1 and 0.9 mm Hg at month 12 observed with the placebo.[30] The development of finerenone represents a critical advancement in the use of non-steroidal MRAs for hypertension.[27]
Two other non-steroidal MRA compounds are making strides: esaxerenone, which has been approved for the treatment of arterial hypertension in Japan, and apararenone, which is currently undergoing clinical trials.[27,31] Esaxerenone is characterized by its high affinity (IC50: 3.7 nM) and exceptional selectivity for MR, with a more than 1000-fold higher selectivity compared to glucocorticoid, progesterone, or androgen receptors. It recently showed promising results in a phase III randomized controlled trial (ESAX-HTN) when compared to eplerenone, demonstrating safety and efficacy in managing hypertension. It also displayed protective effects on the heart and kidneys in hypertensive rats induced by deoxycorticosterone acetate salt, surpassing the results achieved by spironolactone or eplerenone.[22,31] Furthermore, esaxerenone exhibited substantial antihypertensive and renal-protective effects by inhibiting renal inflammatory and oxidative stress pathways, significantly decreasing SBP from 187 ± 6 to 131 ± 4 mmHg.[32] It was also shown to bind to MR, thereby preventing aldosterone binding and MR activation.[33] The results of a long-term phase III clinical trial indicated that esaxerenone can effectively achieve target BP levels either as a monotherapy or in combination with other antihypertensive drugs.[32]
In addition, two more non-steroidal MRAs, AZD9977 (AstraZeneca; Phase I), and KBP-5074 (KBP Biosciences; Phase II), have entered clinical trials. AZD9977 is a novel drug designed to counter the harmful effects of aldosterone and cortisol in non-epithelial tissues while having minimal impact on electrolyte balance in kidney epithelial cells. This tissue-specific modulation suggests that AZD9977 could offer protection against the progression of CV and renal diseases in patients with HFpEF, CKD, or both, without the risk of hyperkalemia associated with steroidal MRAs such as spironolactone and eplerenone.[34] On the other hand, KBP-5074 has demonstrated a significant reduction in SPB in patients with uncontrolled hypertension, with a change in SBP from baseline to day 84 of −15.9 mm Hg in the 0.5 mg cohort and −11.5 mm Hg in the 0.25 mg cohort compared to −5.3 mm Hg in the placebo cohort. In addition, KBP-5074 exhibited a positive impact on urine albumin-to-creatinine ratio reduction in adults with type 2 diabetes and albuminuria, all without inducing serious adverse events related to hyperkalemia.[35,36] These new therapeutic options, including KBP-5074 and AZD9977, may offer a safe approach to controlling hypertension in individuals with hypertension-related complications, without the need for potassium-lowering agents. Without a doubt, research in this field has the potential to significantly contribute to the future development of safe and effective non-steroidal MRAs.
Natriuretic peptide and neprilysin (NEP)
Neurohormonal regulatory mechanisms play a significant role in shaping the pathways leading to HF, primarily acting through three central systems: the autonomic nervous system, the RAAS, and the natriuretic peptide system (NPS).[37] NEP, a zinc-dependent metallopeptidase, has gained recognition as a crucial player in this neurohormonal regulation.[38] It is responsible for breaking down atrial natriuretic peptide, brain natriuretic peptide, bradykinin, and NPS.[22] Consequently, a NEP inhibitor (NEPi) prevents the degradation of vasoactive peptides, including bradykinin, calcitonin gene-related peptide, adrenomedullin, and endothelin-1. NEPi’s beneficial effects encompass vasodilation, enhanced diuretic action, natriuresis, reduced sympathetic activity, and the long-term promotion of anti-inflammatory, antifibrotic, and antihypertrophic effects on cardiomyocytes in vitro.[31] Nevertheless, NEPi alone cannot fully replace existing therapies due to a lack of safety and efficacy data. However, clinical trials have demonstrated the improved prognosis of HF patients and BP reduction in those with uncontrolled hypertension when treated with angiotensin receptor-neprilysin inhibitors (ARNI).[39]
Omapatrilat, the first dual angiotensin converting enzyme (ACE)–neprilysin inhibitor, initially showed significant BP reduction compared to ACE inhibitor monotherapy. However, its synergistic inhibition of bradykinin breakdown led to an increased risk of angioedema, prompting the discontinuation of further research into this drug class.[21] To address this issue, researchers developed LCZ696, a dual ARNI that combines sacubitril and valsartan. LCZ696 has emerged as a treatment for HFrEF. The innovative concept underlying NEP inhibition in HF shifts from complete neurohormonal inhibition to a modulation approach. Sacubitril/valsartan promotes vasodilation, reduces blood volume, and enhances sodium and water excretion through the kidneys by decreasing aldosterone production through its angiotensin receptor blocker-based effects. These mechanisms lead to lowered BP primarily due to reduced blood volume and natriuresis. Sacubitril/valsartan helps balance the neurohormonal environment, offering early relief of HF signs and symptoms and long-term improvements in left ventricular remodeling. These neurohormonal benefits translate into a reduction in major modes of death in HFrEF, including sudden cardiac death and worsening HF.[37] In 2015, the United States Food and Drug Administration (USFDA) approved LCZ696 for the treatment of CV disorders, and it was found to be superior to the angiotensin receptor blocker enalapril in cardioprotection. Ilepatril (AVE 7688) VPi is currently undergoing a phase 3 clinical trial.[31]
Endothelin receptor (ETR) endothelin pathway
Endothelin, a 21-amino acid peptide, is generated by the vascular endothelium from a 39-amino acid precursor called big endothelin. This conversion is facilitated by an enzyme known as endothelin-converting enzyme located on the endothelial cell membrane.[40] Endothelin serves as a potent vasoconstrictor and smooth muscle mitogen and operates through endothelin A and endothelin B receptors.
Patients with pulmonary arterial hypertension (PAH) exhibit overexpression of endothelin in their lungs and plasma.[41] Endothelin receptor antagonists (ERAs) are a class of potent vasodilators and antimitotic agents capable of specifically dilating and remodeling the pulmonary arterial system. Two types of ETRs are involved: endothelin type A receptors (ETAR) primarily found on vascular smooth muscle cells, promoting vasoconstriction by increasing intracellular calcium, and endothelin type B receptors (ETBR) located on endothelial cells, which stimulate the release of vasodilating agents such as NO and prostacyclin.[41] Therefore, either selectively blocking ETAR alone or non-selectively blocking both ETAR and ETBR together can dilate pulmonary vessels.
Numerous clinical studies have demonstrated that blocking the endothelin system reduces BP in patients with mild-to-moderate hypertension and even in those with resistant hypertension.[42] At present, a variety of ETR antagonists (ETRAs) have been developed and can be categorized into three groups based on their functions: selective ETAR antagonists such as darusentan and ambrisentan, non-selective ETRAs such as bosentan and macitentan, and selective ETBR antagonists.[26] Bosentan, the oldest of the ERAs, has been authorized since 2001. While short-term studies have shown improvements in clinical symptoms in patients with PAH, hemodynamics, and time to clinical worsening, the COMPASS-2 study indicated no significant effect in patients already treated with sildenafil. [40] Darusentan, a selective ETAR antagonist, was explored for its use in resistant hypertension, but results were discouraging as the predetermined coprimary endpoints were not achieved, primarily due to BP measurement methodologies. However, a multicenter, randomized, and double-blind study revealed that darusentan dose-dependently reduced BP in patients with essential hypertension.[22,26] Ambrisentan, a selective ERA antagonist, was found to improve various aspects of patient well-being when administered as monotherapy, and its combination with tadalafil showed favorable effects on exercise capacity and long-term outcomes compared to monotherapy with either substance.[41] No known hepatotoxic effects or clinically relevant drug interactions have been associated with ambrisentan. Macitentan, a novel dual ETAR/ETBR antagonist, demonstrated significant reductions in mean arterial pressure and mean pulmonary artery pressure, surpassing bosentan in hypertensive rats and pulmonary hypertensive bleomycin-treated rats.[22] In the SERAPHIN study, macitentan showed favorable effects in patients with PAH, both when used as monotherapy and in combination with other PAH medications, primarily sildenafil.[43]
Aprocitentan (ACT-132577), a new dual ETA/ETB antagonist, significantly inhibits the binding of ET-1 to both ETAR and ETBR. In a phase 2 dose-finding study in hypertension patients, aprocitentan, administered as monotherapy at doses ranging from 10 mg to 25 mg, provided the most favorable profile by effectively lowering BP with low rates of fluid retention.[44] In the phase 3 precision study, patients with resistant hypertension receiving standardized antihypertensive treatment, including a diuretic, showed that the addition of aprocitentan led to reductions in both standardized automated office and 24-h ambulatory BP compared to a placebo after four weeks of initial treatment. Aprocitentan demonstrated a significant decrease in SBP in comparison to the placebo, registering a mean change of −15.3 mmHg for the 12.5 mg dose, −15.2 mmHg for the 25 mg dose, and −11.5 mmHg for the placebo.[44] These findings suggest that targeting ETRs may have therapeutic effects in the treatment of hypertension.
DIABETES AS A RISK FACTOR
Diabetes mellitus (DM) encompasses a range of metabolic disorders characterized by the presence of excessive glucose in the bloodstream, stemming from insufficient insulin production by beta cells (T1DM) or the body’s inability to use insulin effectively (T2DM).[45] The prevalence of DM is escalating globally, leading to various adverse micro- and macrovascular consequences, such as retinopathy, nephropathy, neuropathy, atherosclerosis, and coronary heart disease (CHD).[46] Widely recognized as a significant risk factor, DM significantly contributes to the development of HF and amplifies mortality rates among HF patients.[47]
Recent studies highlight the coexistence of HF and DM, with a prevalence reaching up to 40%, underscoring the imperative need for a deeper understanding of shared pathophysiological pathways and enhanced therapeutic interventions for both conditions.[48] Notably, research published in the American Journal of Cardiology found that individuals with DM are at a two to five times greater risk of developing HF compared to those without DM.[49] Furthermore, when HF is present in individuals with DM, the prognosis is notably unfavorable.[50] For instance, this study reported that individuals with both DM and HF face a tenfold increase in mortality compared to those without DM who have HF. One can readily observe that a significant proportion of people with HF, especially the elderly, have a history of either short-term or long-term DM. This highlights the substantial risk that DM poses to heart health, with HF being the ultimate consequence if this factor is not effectively managed.
In addition to the heightened risk of developing HF, DM exerts other detrimental effects on the CV system. One such effect is the development of insulin resistance and glucose intolerance, conditions frequently observed in HF patients. These conditions, characteristic of DM, can exacerbate HF. Moreover, DM has been linked to the development of diabetic CMP, a condition that impairs myocardial function and renders the heart more susceptible to ischemia while hindering its ability to recover.[49] In addition, diabetes-related autonomic neuropathy can also play a role in the development and progression of HF, with research indicating that diabetic autonomic neuropathy leads to an imbalance in the sympathetic and parasympathetic nervous systems, increasing the risk of CV mortality.
The relationship between DM and HF is further substantiated by findings from the Framingham study,[51] which demonstrated that DM is a significant risk factor for HF, irrespective of the presence of other CV conditions such as CHD and hypertension. At each biennial examination, the study reported an evident excess risk of congestive HF in participants with DM, spanning various ages and genders. Therefore, it is evident that DM exerts a profound influence on the development and prognosis of HF.[47,52] The influence of DM HF development is substantial. Not only does DM raise the risk of developing HF, but it also worsens the outlook for individuals dealing with both conditions. Prolonged exposure to elevated blood glucose levels can harm the blood vessels of the heart and other organs, contributing to various health complications, including HF. The duration of DM correlates with the heightened risk of cardiac issues. Elevated sugar levels lead to atherosclerosis by modifying lipid levels, decreasing high-density lipoprotein, and increasing low-density lipoprotein levels. Epidemiological studies have established the link between HF and DM, highlighting that impaired glucose tolerance, elevated blood glucose levels, and glycated hemoglobin (Hb1Ac) levels are associated not only with systolic HF but also with diastolic dysfunction.[46]
Diabetes is associated with both macrovascular (involving large arteries like conduit vessels) and microvascular (involving small arteries and capillaries) disorders.[53] Chronic hyperglycemia and insulin resistance play pivotal roles in initiating diabetic vascular complications, involving mechanisms like increased formation of Advanced Glycation End-Products (AGEs) and activation of the Receptor for Advanced Glycation End-Products (RAGE) AGE-RAGE axis, oxidative stress, and inflammation.[54]
Macrovascular Disease: Macrovascular (or CVD) disease within the major conduit arteries is a multifaceted inflammatory process that can lead to conditions such as MI, stroke, and peripheral artery disease.[53] Diabetes can induce macrovascular disorders by compromising the inner lining of blood vessels, leading to the accumulation of cholesterol and other fats on artery walls, forming plaques. Over time, these plaques can constrict or obstruct arteries, restricting blood flow to various organs, including the heart, which can eventually lead to HF if not effectively managed. Diabetes can also trigger systemic inflammation, contributing to blood vessel damage. In addition, it can increase the risk of blood clot formation, which can obstruct blood vessels and pose a threat to heart health.
Recent progress in the management of diabetes and its impact on HF
Diabetes has emerged as a global epidemic, with the number of individuals affected continuously on the rise. Notably, it is a well-recognized risk factor for the development of HF and other CVDs. Recent advancements have been made to enhance diabetes management, with the aim of mitigating the potential complications associated with poorly controlled DM. Individuals with DM who develop HF confront a challenging prognosis.[50] Research has consistently highlighted DM as a pivotal risk factor for HF, indicating that individuals with both conditions fare worse compared to those without DMs.[52]
The T2DM exhibits distinctive epidemiological patterns, with its prevalence steadily rising in industrialized nations such as the United States and Japan, as well as in developing countries at an alarming rate.[55] Patients with T2DM are more susceptible to various short- and long-term complications, with T2DM, in particular, having a notable impact on cardiac diseases. When HF develops in individuals with DM, it results in a tenfold increase in mortality and a mere 12.5% five-year survival rate, which is more unfavorable than the prognosis for metastatic breast cancer.[50] In addition, HF patients face an increased risk of developing insulin resistance, glucose intolerance, and DM.
Nevertheless, recent advancements in DM management have shown promise in improving outcomes for individuals afflicted by both DM and HF. A significant breakthrough lies in the development of medications specifically targeting CV risks associated with DM. These innovations encompass new drug formulations, continuous glucose monitoring (CGM), bionic pancreas, stem cell therapy, gene therapy, and the integration of artificial intelligence (AI), among others. For instance, SGLT-2 inhibitors have demonstrated a remarkable reduction in HF hospitalizations among individuals with T2DM, irrespective of existing CVD. Another notable development is the implementation of CGM systems, providing real-time insights into blood glucose levels, and empowering individuals with diabetes to make informed decisions regarding medication dosages and lifestyle choices.
New medication development
Newer drugs have been developed to better manage DM. The addition of a new DM medication does not diminish the need of making dietary changes and improving physical exercise. However, if the usage of first-line treatments (e.g., metformin), adjustments to one’s diet, and exercise are insufficient to get one into adequate blood sugar management, then using one of the new medications can prevent or lessen the issues that emerge when one lives with diabetes. These newer medications include dipeptidyl peptidase-4 (DPP-4) inhibitors, glucagon-like peptide-receptor agonists (GLP-1RAs), and SGLT-2 inhibitor.
DPP-4 inhibitors and HF
DPP-4 inhibitors are favored for managing T2DM due to their tolerability and the convenience of oral dosing.[53] However, in patients with established CVD or metabolic issues, the use of DPP-4 inhibitors, particularly saxagliptin and alogliptin, may exacerbate HF.[56] Although some studies suggest a link between DPP-4 inhibitors and HF, others do not, and some studies have made corrections to their findings. For instance, the SAVOR-TIMI 53 randomized trial found that the DPP-4 inhibitor saxagliptin increased the incidence of HF hospitalizations in patients with established CVD or multiple CV risk factors, particularly within the first 12 months of medication.[56] Although, the risk associated with saxagliptin treatment was highest in patients at a high overall risk of HF, as indicated by factors such as a history of HF, impaired renal function, or elevated baseline levels of NT-proBNP, and significantly lower in patients at lower risk.[56]
Similarly, the EXAMINE trial found that alogliptin, another DPP-4 inhibitor, had similar outcomes to placebo in individuals with T2DM and a recent acute coronary syndrome incident.[57] In individuals with a history of HF at baseline, subgroup analyses showed that alogliptin did not result in more new HF hospital admissions or poorer outcomes for existing HF.[57] In addition, Mcmurray et al. reported findings related to vildagliptin, demonstrating that it was not inferior to placebo in terms of changing left ventricular ejection fraction (LVEF). However, treatment with this DPP-4 inhibitor did reveal an increase in left ventricular volumes.[58] Importantly, there was no observed increase in BNP levels or any other evidence suggesting a deteriorating HF condition.[58] These findings are inconclusive regarding whether DPP-4 inhibitors directly cause or increase the risk of HF in T2DM patients, indicating the need for further research. In the meantime, it is important to note that DPP-4 inhibitors remain FDA-approved for the management of T2DM.
Glucagon-like peptide-receptor agonists (GLP-1RAs) and HF
GLP-1RAs, also referred to as GLP-1 agonists, incretin mimetics, or GLP-1 analogs, stimulate the release of insulin by mimicking the action of incretin. Notable drugs in this category include dalutide, exenatide, liraglutide, lixisenatide, semaglutide, and tirzepatide. The GLP-1 hormone triggers insulin production, inhibits the release of glucagon, delays stomach emptying, and enhances feelings of fullness. By curbing glucagon secretion, these agents prevent an excess of glucose from entering the bloodstream. Slowing down the digestive process reduces the amount of glucose released. Consequently, GLP-1RAs bind to the GLP-1 receptor and initiate these effects.
The effects of GLP-1-RAs on HF in individuals with DM have generated ongoing debate. In the LEADER trial, the administration of liraglutide to individuals with T2DM at high CV risk significantly reduced the incidence of death from CV causes, non-fatal MI, or non-fatal stroke when compared to a placebo.[59] However, liraglutide did not demonstrate superiority over placebo in preventing hospitalizations due to HF (HHF) among patients with both T2DM and high CV risk.[59] Similar findings were observed in trials such as SUSTAIN-6, ELIXA, EXSCEL, and the REWIND trial, where clear reductions in HF risk were not evident.[60]
In the Harmony Outcomes trial, which involved patients with T2DM and established CV disease, the use of albiglutide was associated with a non-significant 15% decrease in the risk of a combined outcome involving death from CV causes or HHF (heart rate [HR] 0.85, P = 0.113).[61] Nevertheless, albiglutide did show a significant 29% reduction in the risk of incident HHF compared to a placebo, particularly among the subgroup of patients with a history of HF at baseline.[61] The impact of albiglutide on the composite outcome of CV death or HHF was more pronounced in patients without prior HF (HR 0.73, 95% CI: 0.56–0.95).[61] In addition, a meta-analysis focusing on the effects GLP-1RAs on HF was conducted. The collective findings demonstrated that GLP-1RAs could reduce the risk of hospitalization for HF and improve the performance in the 6-min walk test in individuals with no prior history of HF. However, the impact of GLP-1RAs on HF hospitalization in individuals with a history of HF, levels of NT-proBNP, and quality of life (QoL) scores were not deemed statistically significant. Furthermore, GLP-1RAs did not exhibit a significant effect on LVEF, but they did lead to a significant decrease in E-wave velocity, E/A ratio, E/e’ ratio, and E-wave deceleration time.[62] These findings collectively suggest that while GLP-1RAs may not directly enhance HF prognosis, they may hold potential clinical benefits related to ventricular diastolic function. It is worth noting that several systematic reviews have acknowledged the potential for GLP-1RAs to improve hospitalization rates for HF, extending this benefit even to individuals without diabetes.[62]
SGLT-2 inhibitors and HF
SGLT-2 inhibitors, a class of antihyperglycemic medications, have shown remarkable and early advantages in preventing HF and CV mortality in high-risk T2DM.[63] Notable drugs in this category include canagliflozin, dapagliflozin, empagliflozin, ertugliflozin, and bexagliflozin. Recent CV outcome studies mandated by the USFDA have revealed that both empagliflozin and canagliflozin were associated with a remarkable 35% reduction in hospitalizations due to HF among participants with established CV or CV risk factors.[64] The early and pivotal role of changes in metabolic flexibility and substrate utilization in HF development is well-established, leading to unfavorable remodeling and progression to severe HF in experimental and clinical contexts.
SGLT-2 inhibitors are known to boost the production of ketone bodies, including ß-hydroxybutyrate (ßOHB). These benefits are attributed to the ability of SGLT-2 inhibitors to boost the production of ketone bodies, such as ßOHB. It is hypothesized that this increase in myocardial ketone body oxidation may enhance cardiac adenosine triphosphate production, serving as a preferred energy source over fatty acids or glucose in the diabetic heart.[64] Ketone bodies have been identified as a vital energy source in hypertrophied and failing hearts, and the intricate interplay between ketone bodies, glucose, and fatty acid oxidation, particularly concerning SGLT-2 inhibitors, remains a subject of ongoing investigation.[65]
SGLT-2 inhibitors have emerged as valuable therapeutic tools in the management of T2DM, offering protection against cardiac diseases and significantly benefiting patients with HF. These inhibitors exhibit cardioprotective and renoprotective effects across various conditions, encompassing T2DM, CKD, and HF. In individuals with HF, their clinical benefits are most pronounced in those with reduced ejection fraction and are now strongly recommended as a pivotal component of comprehensive disease management.[66]
Clinical trials have unveiled the potential CV advantages of SGLT-2 inhibitors that extend beyond glycemic control. A systematic review and meta-analysis conducted by Butler et al. in 2017, involving nearly 17,000 HF patients, indicated substantial reductions in all-cause mortality, CV mortality, HF hospitalizations, and renal complications with the use of SGLT-2 inhibitors.[23] Studies like the SCORED and SOLOISTWHF trials further emphasized the benefits of sotagliflozin, a dual SGLT-1/SGLT-2 inhibitor, in reducing the risk of CV deaths and hospitalizations for HF in patients with T2DM combined with CKD or recent worsening HF.[67] A growing body of clinical trials, including EMPA-REG OUTCOME, CANVAS Program, CREDENCE, and DECLARE-TIMI, have affirmed that SGLT-2 inhibitors not only provide kidney protection but also confer significant CV benefits.[67]
In patients with varying degrees of HFrEF, treatment with empagliflozin or dapagliflozin, when added to the appropriate HF management, resulted in reduced all-cause and CV mortality, fewer hospitalizations for HF, and fewer serious renal complications.[57] The DAPA-HF trial demonstrated that dapagliflozin effectively lowered the risk of HF progression and CV-related deaths in individuals with HFrEF (New York Heart Association [NYHA] class II–IV), irrespective of their glycemic status or gender.[59] Moreover, the EMPEROR-preserved trial presented the initial evidence of empagliflozin’s cardioprotective effect on the combined risk of hospitalizations for HF and CV mortality in individuals with HFpEF, regardless of their diabetes status.[59] The SGLT-2 inhibitors have recently been recommended as fundamental therapy for HFrEF, and early initiation is advised to swiftly enhance clinical outcomes and QoL.[68] It is important to acknowledge that while SGLT-2 inhibitors offer substantial benefits, certain side effects warrant attention, such as genital and urinary tract infections, diabetic ketoacidosis, and bone fractures.[69,70]
Continuous glucose monitoring
Effective glucose monitoring is the cornerstone for mitigating acute and chronic complications, reducing disability, and preventing premature mortality in individuals with DM.[71] Maintaining proper glycemic control in diabetic patients significantly reduces the risk of developing CV conditions, including HF, as a consequence of DM as a risk factor. Conventionally, monitoring glycemic levels has relied on the average of HbA1c levels over 2–3 months. However, HbA1c may not be a suitable marker for patients with abnormal hemoglobin, end-stage renal illness, or chronic liver disease.[61] In addition, HbA1c cannot predict daily fluctuations in glucose levels, such as episodes of hypoglycemia.[72] Therefore, patients are required to perform self-monitoring of blood glucose (SMBG) to assess daily variations in blood glucose, set individualized glycemic targets, and tailor their DM management accordingly.[72] However, SMBG has limitations in providing a comprehensive overview of blood glucose patterns and detecting hyperglycemic events.[73] To address these limitations, CGM with wearable devices has emerged as a method for customizing treatment plans with the aim of enhancing glycemic control.[74] Patients with DM who utilize CGM experience more substantial reductions in Hb1Ac, body weight, caloric intake, and exhibit greater adherence to dietary and physical activity recommendations compared to those using standard SMBG.[75] These improvements contribute to a reduction in the risk of complications that may lead to HF.
The daily glucose data generated by CGM enable patients to comprehend the connections between self-care, medication adherence, nutrition, physical activity, and glycemic management.[74] CGM empowers patients to monitor their glucose levels, make informed decisions about medical consultations, track their dietary choices, identify abnormal readings, and determine the factors causing them, allowing for timely adjustments. Clinicians and nutritionists, in turn, utilize CGM information to optimize medication and nutrition-based interventions and educate patients on self-care practices, aided by clear and patient-friendly summaries of glucose patterns and profiles.[72] In comparison, routine blood glucose measurement methods, such as HbA1c tests and patient SMBG, lack the ability to capture glucose fluctuations.[72]
Bionic pancreas
The use of insulin pumps, including commercially available hybrid closed-loop systems that offer partial automation of insulin delivery, typically involves inputting various parameters such as basal rates, insulin-sensitivity factors, carbohydrate-to-insulin ratios, total daily insulin doses, or a combination of these factors.[76] At mealtimes, insulin doses are determined by the user entering the number of grams of carbohydrates in the meal, and effective treatment may depend on user-initiated correction doses for hyperglycemia.[65] These systems may also require a warm-up period during which the user’s insulin dosing data is incorporated before full automation can begin.[76]
In contrast, the iLet bionic pancreas developed by Beta Bionics operates differently. It does not rely on the patient’s previous insulin regimen, such as basal and bolus dose settings. Instead, it is initialized solely based on the patient’s body weight. This bionic pancreas fully automates the calculation and delivery of all required insulin doses immediately after inputting body-weight data, without the need for a warm-up period.[76] A multicenter randomized trial led by Russell et al. assessed the use of a bionic pancreas in individuals with type 1 diabetes. The trial showed that using the bionic pancreas in an insulin-only configuration was associated with reduced levels of Hb1Ac, lower mean glucose levels, an additional 2.6 h/day spent within the target glucose range, and less time spent in a hyperglycemic state, all without an increase in the incidence of hypoglycemia.[76] Notably, this device has received approval from the USFDA and is currently being used by some individuals with type 1 diabetes. Based on the promising results from Russell’s research and his team, the beta bionic pancreas is a promising tool that can help reduce the risk of HF associated with diabetes as a risk factor.
Stem cell therapy
Therapeutic approaches for controlling hyperglycemia in DM, such as exogenous insulin and other hypoglycemic medications, have limitations as they only delay the onset of diabetes-related complications and do not replicate the natural secretion of insulin, leading to unstable glucose levels and disruptions in patients’ daily lives.[77]
Stem cells are characterized by their unique ability to undergo self-renewal, generating additional cells, and providing daughter cells that can differentiate into specific cell lineages.[78] With immunomodulatory properties, the capacity to differentiate into specialized cells, and the potential for regeneration, stem cells present a promising and novel therapeutic avenue for addressing diabetes and its complications.[77] These unspecialized cells possess the remarkable capability of both self-renewal and differentiation into mature cells with specific functions.[79] Stem cells exhibit the capacity to differentiate into various cell types, including heart muscle cells, making them a viable treatment option. They can be categorized based on their origin, with common sources including bone marrow, adipose tissue, or even the cardiac tissue itself.[78]
In a study conducted by Bhansali et al., stem cells were harvested from autologous bone marrow retrieved through the posterior superior iliac spine route under local anesthesia.[79] These stem cells were then injected into the gastroduodenal artery using a 5F catheter navigated through the transfemoral route.[79] The study’s primary endpoints after 6 months of stem cell transplantation (SCT) included a 50% reduction in insulin requirement and an improvement in glucagon-stimulated C-peptide levels.[79] The results demonstrated that SCT reduced the daily insulin dosage needed by 70% of patients after just one treatment, improved glucagon-stimulated C-peptide levels, and lowered HbA1c by 1.1%.[79] Numerous other studies have reported successful outcomes with stem cell therapy in diabetes management, indicating its potential to rejuvenate the pancreas’s natural insulin production. Administered in the early stages, stem cell therapy may reverse dependency on medications and insulin, consequently reducing the risk of diabetes-related complications, including HF.
Gene therapy
Gene therapy, a nascent field of medical research, focuses on manipulating human cells’ genetic material to treat or potentially cure specific diseases. This approach involves repairing or replacing faulty genetic material within the body.
In the context of type 1 diabetes, researchers are reprogramming alternative cells to perform the functions of beta cells, including insulin production. Using an adeno-associated viral (AAV) vector, researchers like Xiao et al. in 2018 transferred two proteins, pancreatic and duodenal homeobox 1, and musculoaponeurotic fibrosarcoma (MAF) basic leucine zipper transcription factor A, to a mouse’s pancreas. This intervention transformed alpha cells to function similarly to beta cells, leading to normal blood sugar levels in mice for up to 4 months after gene therapy, all without the need for immunosuppressive drugs that restrict or halt immune system activity.[80] Although there are limited studies, primarily focusing on in vivo experiments, exploring the use of gene therapy for managing type 1 diabetes, its potential contribution to preventing HF complications in diabetes remains uncertain due to its early stages of development.
Potential therapeutic approaches targeting diabetes-related HF
The combination of SGLT-2 inhibitors with plant extracts, such as Cassia angustifolia, Morus alba, Cinnamonium cassia, and Rauwolfia serpentina, is proposed for a synergistic effect in addressing diabetes-related HF. Some plant extracts have demonstrated mechanisms to counteract insulin resistance. For instance, C. angustifolia extract has been shown to increase GLUT-4 expression and turnover through the G protein-PLC-PKC signaling pathway and the inositol 1,4,5-triphosphate receptor.[81] Similarly, Morus alba L. leaf extract has been found to reduce insulin resistance and enhance glucose metabolism at the muscle level by activating the IRS-1/PI3K/Glut4 signaling pathway.[82] Overall, the combination of SGLT-2 inhibitors and specific plant extracts presents a promising approach to address the complex metabolic disruptions in cardiac tissue associated with DM.
The use of mechanical circulatory support (MCS) devices
Heart transplantation is considered the definitive treatment for individuals with refractory end-stage HF, the demand for donor hearts exceeds availability. Consequently, MCS has become crucial in treating patients with end-stage HF.[83]
In the 1960s, Drs. Michael DeBakey and Frank Spencer recognized the benefits of cardiopulmonary bypass (CPB) extending beyond the operating theater into the post-surgical recovery period. Resting the heart on CPB for extended periods allowed for ventricular function recovery in patients with cardiogenic shock.[84] VADs, a type of MCS device, have shown significant positive impacts in HF management. Various types of VADs include the left VAD (commonly fixed at the left side of the heart to pump oxygenated blood to the body), right VAD (pumping deoxygenated blood from the right side of the heart to the lungs), biventricular assist device (supporting both sides of the heart), and pediatric VAD (used for individuals ranging from newborns to young adults). The implantation of VADs into the hearts of patients requiring this device involves close monitoring to ensure proper functionality before hospital discharge.
Positive experiences with adult MCS suggest that, for appropriate pediatric-age patients not expected to rapidly recover sufficient ventricular function or facing prolonged transplantation waiting times, implantable VADs provide the most beneficial form of chronic mechanical support.[85] Complications associated with MCS, such as infection, bleeding, and thrombosis, have been reported.[83] While these complications are not universal in every VAD procedure, further studies are necessary to explore ways to prevent or manage them if they arise. At present, VADs serve as life-saving interventions for HF patients.
COMBINED APPROACHES
Patients with HF often exhibit multiple coexisting risk factors such as hypertension, cardiac hypertrophy, and DM, further complicating their care. Addressing these risk factors individually may not be sufficient, prompting an increased interest in exploring synergistic therapies that concurrently target multiple aspects of HF’s underlying pathophysiological mechanisms and the interplay between different risk factors.
One avenue for synergistic therapy involves the use of combination drug therapies. These combinations entail administering multiple medications simultaneously, each targeting different facets of HF, including hypertension, cardiac hypertrophy, and fluid retention. Combining therapies that address these aspects simultaneously has shown promise in improving outcomes for HF patients. For instance, a combination of ACE inhibitors, beta-blockers, and diuretics may be employed to address hypertension, cardiac hypertrophy, and fluid retention concurrently, leading to improved symptoms, reduced hospitalizations, and extended survival. However, the varying diuretic doses needed based on individual response, fluid status, and renal function can complicate precise adjustment and titration of combination therapy, necessitating close monitoring and frequent adjustments for optimal outcomes. Furthermore, when HF coexists with DM and CKD, the prognosis becomes notably challenging which is worse than many cancers.[86] The relationship between diabetes and HF involves not only complications from ischemic heart disease but also metabolic disorders such as glucose toxicity, lipotoxicity based on insulin resistance, vascular endothelial dysfunction, microcirculatory disorder, and capillary failure.[53,87] Achieving glucose control in DM is primarily aimed at avoiding microvascular complications, and the correlation between glycemic control and CV outcomes involves complex interactions among various factors.
The treatment of HF is generally similar for patients, whether they have diabetes or not. However, antidiabetic drugs can have varying effects in individuals with HF, underscoring the importance of prioritizing medications that are safe and can reduce HF-related events.[87] Certain glucose-lowering therapies have demonstrated potential benefits in patients with HF and other CV conditions.[88] Recent trials have investigated the impact of these therapies on mortality in HF patients, offering insights into their potential risks and protective effects in the context of diabetes. Antihypertensive drugs, including ACE inhibitors, angiotensin-receptor blockers, mineralocorticoid-receptor blockers, and calcium-channel blockers, may exert direct vasoprotective effects. Their use may contribute, at least in part, to the reduction of vascular complications in patients with diabetes and concomitant hypertension. Tight control of BP has been demonstrated to decrease CV risk in T2DM, with recent guidelines from the US and Canada recommending a target BP of <130/80 mm Hg.[53] ACE inhibitors are currently recognized as the first-line antihypertensive therapy in diabetic individuals with proteinuria. These agents offer unique benefits in modifying the progression and severity of CVD as well as diabetic nephropathy.[89]
Emerging therapeutic approaches are currently under development to address CV complications associated with diabetes, focusing on oxidative stress, inflammation, and fibrosis.[90] Specifically, drugs that enhance Nrf-2 activity, such as bardoxolone methyl, and strategies to inhibit the NLRP3 inflammasome, are being explored for their therapeutic potential. A derivative of bardoxolone methyl, dh404, has demonstrated efficacy in attenuating endothelial dysfunction, reducing Nox1 expression, lowering oxidative stress, and inhibiting inflammation in diabetic mice. This suggests that the upregulation of Nrf2 may have therapeutic potential in limiting vascular damage associated with diabetes.[91]
In addition to combination drug therapies, there is a growing interest in the use of antioxidants as synergistic treatments for HF. Oxidative stress and mitochondrial dysfunction play pivotal roles in the development and progression of HF. Utilizing antioxidants, such as Vitamin C and Vitamin E, is considered a strategy to counteract oxidative stress and enhance cardiac function in HF patients. Various studies suggest that antioxidant therapy, including drugs like tempol, may be effective in preventing and treating HF.[92] However, it is crucial to note that randomized clinical trials have not consistently demonstrated the benefits of antioxidant supplementation in reducing CVD outcomes. Despite this, the use of antioxidants in conjunction with other therapies targeting multiple risk factors shows promise in the management of HF.[93]
Clinical trials and experimental models investigating multi-agent therapies
Recent research, conducted through clinical trials and experimental models, has yielded promising results in evaluating combination therapies aimed at addressing multiple risk factors concurrently, including hypertension, DM, and cardiac hypertrophy, for the treatment or prevention of HF. Studies have indicated the effectiveness of combining ACE inhibitor therapy with diuretics in reducing left ventricular hypertrophy, a common risk factor for HF.[94] In addition, the combination of an ACE inhibitor and an AT 1 receptor antagonist has demonstrated the ability to lower BP in normotensive individuals and improve cardiac dysfunction in HF patients.[95] These findings suggest that combining different therapeutic agents may be more effective in treating multiple risk factors associated with HF compared to using a single agent alone.
Further evidence from clinical trials has shown that the combination of CI-928 and CI-914 provides synergistic and beneficial effects in an acute HF model.[96] Furthermore, ACE inhibitor therapy alone has been shown to produce prolonged clinical improvement in patients with moderately severe congestive HF. Guidelines for clinical practice also recommend a combination of ACE inhibitors, diuretics, and digoxin for the treatment of HF.[97]
Clinical trials and experimental models have provided compelling evidence for the efficacy of combination therapies in addressing multiple risk factors associated with HF. These studies have shown that combining different therapeutic agents, such as ACE inhibitors, diuretics, and AT 1 receptor antagonists, can have synergistic effects and improve outcomes in patients with HF. Future research should focus on further investigating the optimal combinations and dosages of these therapeutic agents, as well as identifying specific patient populations that would benefit the most from combination therapy. The demonstrated efficacy of combination therapies in concurrently addressing risk factors such as hypertension, diabetes, and cardiac hypertrophy underscores their potential in treating or preventing HF. These findings highlight the potential of combination therapies to synergistically target different pathways involved in HF pathogenesis, leading to improved outcomes.
Potential benefits of a multifaceted approach to HF prevention
The potential advantages of adopting a multifaceted approach to HF prevention are substantial and could significantly impact the health-care system. Research suggests that successfully achieving multiple pharmacological and lifestyle targets for HF prevention may have a multiplicative effect, similar to the risks associated with multiple risk factors.[98]
Studies have illustrated the effectiveness of multidisciplinary approaches to managing HF, supported by meta-analyses and best practice guidelines recommending specialist HF disease management programs.[99] These programs typically involve comprehensive and multidisciplinary interventions tailored to individuals with HF or those at high risk, encompassing elements such as medication management, lifestyle modifications, education and counseling, exercise programs, and support from healthcare professionals and caregivers. Implementing these multifaceted approaches makes it challenging to pinpoint the incremental benefits of each intervention component.
Lifestyle modification stands out as a crucial means to prevent atherosclerotic vascular disease, HF, and atrial fibrillation, even though evidence supporting the utility of such changes is limited. However, there are multiple barriers to implementing lifestyle changes in real-world clinical practice.[100] Stage A HF, or “pre-HF,” refers to patients at high risk for developing symptomatic HF, with risk factors including atherosclerotic heart disease, hypertension, and diabetes.[101] Despite aggressive efforts to achieve BP, glucose, and lipid goals through pharmacological approaches, the prevalence of HF continues to rise, suggesting that focusing on the upstream drivers (i.e., lifestyle factors) of individual risk factors might yield incremental benefits. Quitting smoking and maintaining a healthy weight are emphasized as essential steps for heart health, as smoking cessation significantly reduces CV risk, and obesity contributes to strain on the heart and risk factors for HF.
Evidence also suggests that a small reduction in CV risk across a large population may be more effective in preventing CV events than treating only a small number of individuals with high risk. Multifaceted approaches to HF prevention have the potential to address multiple risk factors simultaneously, leading to a greater overall reduction in CV risk. This approach has proven effective in preventing hospital readmissions, particularly for elderly adults with chronic HF.[102] In addition, a multifaceted approach to HF prevention allows for individualized care, tailoring interventions to meet the specific needs and preferences of patients and their health-care resources. Moreover, the combination of interventions in a multifaceted approach may enhance motivation and self-management skills among patients, resulting in a reduction in the risk of all-cause mortality and acute HF.[103]
INNOVATIVE THERAPIES
In an era defined by transformative technological breakthroughs across diverse facets of our existence, it is unsurprising that the health-care system is also undergoing a transformative shift. Notably, advancements in technology are playing a pivotal role in reshaping CV care and preventing HF. The landscape of heart health is evolving with the introduction of novel medications, state-of-the-art monitoring technologies, and innovative device therapies, marking a departure from historically limited treatment options.[104] These cutting-edge technologies and therapies aim to prevent the development and progression of HF, thereby enhancing patient outcomes and overall QoL.
A pivotal advancement in the prevention of HF revolves around the development of groundbreaking medications. Complementing this, monitoring technologies have ushered in a paradigm shift in heart health surveillance, playing a pivotal role in prevention. These technologies allow for continuous monitoring of key parameters such as BP, HR, and fluid status, and empowering healthcare providers with real-time data for informed decision-making and early intervention in cases of deteriorating HF.
In recent times, innovative technologies and therapies include implantable devices such as cardiac resynchronization therapy devices, implantable cardioverter-defibrillators,[105] demonstrate the continuous efforts to improve outcomes for patients with HF by providing new pathways for prevention, intervention, and treatment. For instance, implantable devices such as cardiac resynchronization therapy devices and implantable cardioverter-defibrillators offer real-time cardiac function monitoring and timely delivery of therapeutic interventions, such as pacing or defibrillation, when necessary. Furthermore, advancements in analytics and biomarker research have illuminated the molecular pathways associated with HF, with cardiac resynchronization therapy (CRT) showcasing the potential to restore normal contraction patterns, significantly improve patient QoL, reducing the risk of hospitalization and increasing survival.[106] Among patients with NYHA class II or III HF, a wide QRS (part of electrocardiographic wave) complex, and left ventricular systolic dysfunction, the addition of CRT to implantable cardioverter-defibrillators reduced rates of death and hospitalization for HF.[107]
Another groundbreaking development in heart health monitoring lies in the application of AI algorithms. These algorithms analyze extensive datasets from diverse sources, including wearable devices and medical records, to identify patterns and predict HF risks. The implications of AI extend to diagnosing CV diseases, predicting outcomes, optimizing treatment strategies, and uncovering patterns imperceptible to the human eye. For instance, machine learning algorithms trained on large datasets accurately identify electrocardiogram patterns, aiding in the diagnosis of arrhythmias or ischemic events. AI also plays a pivotal role in analyzing cardiac imaging data, such as echocardiograms or cardiac magnetic resonance imaging scans, to evaluate cardiac structure and function, and identify abnormalities.[108] These predictive capabilities empower health-care providers to intervene early and implement preventive measures, ultimately saving lives and improving patient outcomes.
The rise of wearable devices, including smartwatches and fitness trackers, has gained popularity among individuals seeking to actively manage their heart health. These devices facilitate continuous monitoring of health status, offering timely intervention opportunities. Although standalone wearable devices may have a limited impact on health behavior change, their effectiveness is amplified when combined with targeted behavior change strategies.[109] Equipped with sensors to track HR detect irregularities, and provide feedback on physical activity levels, these devices can issue alerts in the presence of abnormalities, enabling individuals to seek medical attention promptly. Integrating wearable devices into electronic health records and data analytics systems is crucial for leveraging remote monitoring data effectively. This integration facilitates seamless communication between patients and health-care providers, enabling remote consultations, data review, and virtual follow-up visits. However, challenges related to data security, interoperability, and information overload must be addressed to ensure the successful integration of wearable devices into routine clinical practice.[108]
Nanotechnology introduces exciting possibilities in cardiology, particularly in targeted drug delivery and diagnostics. Nanomedicine is designed to identify specific sites in the CV system, delivering medications, diagnostics, and therapeutics with the aim of minimizing side effects and maximizing therapeutic effects.[110] Notably, the delivery of the adenoviral S100A1 gene normalized S100A1 protein expression in a post-infarction rat model of HF has shown promise in restoring protein levels and function in failing myocardium, presenting a novel therapeutic strategy for HF treatment.[109] Targeted nanoparticles designed to bind specifically to desired proteins or biomarkers show promise in detecting early-stage HF with sensitivity and accuracy, enhancing the prospects of successful treatment and potentially leading to a cure.[107]
Telemedicine and remote patient monitoring have also emerged as game-changers in heart health monitoring. With telemedicine, patients can have virtual consultations with their health-care providers, reducing the need for in-person visits. Remote patient monitoring allows healthcare providers to track patients’ vital signs, such as BP and HR, from a distance. This technology ensures proactive management of heart health and early detection of any concerning changes.
Innovative therapies for preventing HF
Beyond the realm of advanced monitoring technologies, pioneering therapies are playing a pivotal role in the prevention of HF. These innovative interventions show tremendous promise in the repair and regeneration of compromised cardiac tissue, holding immense promise in mitigating the underlying causes of heart disease through precision-targeted treatments. Within this field, diverse groundbreaking strategies, such as gene therapy, stem cell therapy, and personalized medicine, are ushering in a new era of possibilities.
Gene therapy
In the context of gene therapies for HF, various transgenes with specific intended impacts are considered, including the regulation of intracellular calcium. This involves the introduction of genes that encode proteins crucial for cardiac function or the silencing of genes that contribute to disease progression. For example, a study published in the journal Circulation Research demonstrated that gene therapy targeting the SERCA2a gene, which plays a key role in regulating calcium ion balance in cardiac cells, has shown improvement in heart function among HF patients.[111] SERCA2a serves as a calcium cycling regulator within the heart, primarily function involves facilitating the relaxation of cardiac muscle by sequestering calcium ions in the sarcoplasmic reticulum. This regulatory mechanism is fundamental in maintaining the balance of calcium levels, a key factor in the contraction and relaxation cycles of cardiac muscle.[112] Despite promising results in early trials, further research is essential to fully comprehend the safety and efficacy of these approaches.
A significant characteristic of chronic HF is the dysfunction of the β-adrenergic receptor (βAR), with G-protein-coupled receptor kinase 2 (GRK-2) playing a disruptive role. This disturbance leads to the downregulation of βARs and a diminished contractile response to adrenergic agonists, both characteristic of chronic HF.[112,113] The β-Adrenergic receptor kinase c-terminal peptide (βARKct), inhibiting endogenous βARK1, has shown promise in preserving cardiac function and delaying HF progression through GRK-2 inhibition.[103] Thus, βARKct when overexpressed improved left ventricular contractility and delays HF progression through GRK-2 inhibition.[114] βARKct cardiac gene transfer contributes to the preservation of cardiac function, demonstrating improvements in the left ventricular contractility in both rodent and large animal models.
Researchers are exploring the use of viral vectors as a means to deliver therapeutic genes directly into heart cells. Viruses can undergo modification to carry the desired genes, serving as efficient carriers for targeted delivery to specific cells. In the early stages, predominantly adenoviral and γ-retroviral vectors were employed. However, recent technical advancements have facilitated the large-scale production of AAV and lentiviral vectors. As of now, adenoviruses and AAVs stand out as the primary viral vectors utilized in clinical trials related to cardiac interventions.[115] Once introduced into the cells, these therapeutic genes possess the capability to rectify faulty genes or provide additional copies of a healthy gene, compensating for the defective counterpart. This approach holds significant promise for addressing the root causes of genetic abnormalities associated with HF. In addition to viral vector-based strategies, there is considerable excitement surrounding RNA-based therapies. This innovative approach involves targeting RNA molecules implicated in HF to develop treatments that specifically tackle the underlying genetic abnormalities. By focusing on RNA, scientists aim to modulate gene expression in a more targeted and precise manner. This avenue not only presents a promising therapeutic approach but also holds the potential for tailoring treatment plans to individual patients, paving the way for personalized and more effective interventions.
Despite the great promise gene therapies hold for HF, substantial challenges exist in translating these therapies to human patients.[111] A major obstacle lies in delivering therapeutic genes efficiently and safely to heart cells. Researchers are actively investigating various delivery methods to optimize gene transfer and minimize the risk of adverse effects. In addition, long-term safety and effectiveness studies are crucial to ensuring the viability of these therapies in clinical practice, addressing potential severe host immune responses, and determining the optimal timing of administration.
Stem cell therapy
In the realm of HF, stem cell therapy involves utilizing these cells to repair and regenerate damaged myocardial tissue, exhibiting promise in regenerating functional heart muscle within degenerated and scarred myocardium.[116,117]
Clinical trials have demonstrated the safety and efficacy of stem cell therapy, leading to its integration into mainstream heart health care. Initial trials, dating back to 2002, targeted the treatment of ischemic HF using stem cells. Key randomized clinical trials such as STAMI, RE. PAIR-AMI, and TOP CARE-CH. D reported conflicting data on stem cell advantages for cardiac function in 2006. However, subsequent trials like STAR-HEAR.T, BOOST, SC. IPIO, ĊADUCEÚS, ŖEGEÑT, and Focus HF demonstrated the positive impact of stem cells on remodeling and the function of ischemic myocardium.[118] For instance, the Focus-HF trial revealed that intramyocardial transplantation of autologous bone marrow mononuclear cells improved QoL and exercise capacity in patients with ischemic HF, attributing these effects to enhanced perfusion of the ischemic myocardium. Similarly, the application of CD34+ stem cells directly into the myocardium of patients with refractory angina demonstrated significant reductions in both the frequency and duration of anginal episodes, as highlighted by Vrtovec et al. in 2013.[119] In the SCIPIO trial, the intracoronary infusion of cardiac stem cells was investigated in patients with ischemic HF who had experienced an acute MI. The results of this trial indicated a notable reduction in infarct size and an improvement in the left ventricular function, underscoring the positive impact of intracoronary stem cell infusion in post-MI patients with HF.
Stem cell therapy for HF has shown promise in both pre-clinical research and early clinical trials, with the first approach aiming to prevent additional damage to the heart post-heart attack, while the second concentrates on fostering the growth of new, healthy heart tissue.[116] Recent advancements highlight the potential of mesenchymal stem cell (MSC) transplantation in impeding further cardiac damage post-MI and promoting the regeneration of functional heart muscle. MSCs, derived from sources such as bone marrow or adipose tissue, release cytokines, and growth factors that activate endogenous repair mechanisms, expediting the healing process. Moreover, MSCs can differentiate into cardiomyocytes when introduced into the murine myocardium, presenting an alternative treatment avenue for individuals with HF.[78,120] Notably, even allogeneic MSCs appear to be non-immunogenic and non-inflammatory, enhancing their appeal as a potential therapeutic option.[121] In addition to MSCs, induced pluripotent stem cells (iPSCs) have emerged as a promising tool for improving heart function post-MI. iPSCs are adult cells that undergo reprogramming to attain a pluripotent state, allowing them to differentiate into heart cells. This innovative technology leverages the patient’s own cells, thereby reducing the risk of rejection or immune-related complications. However, further research is essential to comprehensively understand the mechanisms and refine the delivery methods of stem cell therapy for HF. Ongoing investigations are crucial for advancing the therapeutic potential of these regenerative approaches and establishing their place in the clinical management of HF.
While stem cell treatments offer exciting possibilities, challenges remain, particularly in ensuring the survival and integration of transplanted stem cells within heart tissue. Scientists are actively exploring strategies such as tissue engineering and biomaterial scaffolds to enhance cell retention and integration. In addition, refining the timing and delivery methods of stem cell treatments is crucial for maximizing their therapeutic effects.
Personalized medicine
Precision medicine, also known as personalized medicine, is increasingly influential in the realm of HF prevention and treatment, with recent advancements in medical therapies, monitoring technologies, and device interventions reshaping HF management.[104] While HF remains a formidable and progressive condition, especially in Sub-Saharan Africa, where a substantial burden exists, advanced treatments such as heart transplantation and artificial heart blood pumps face constraints related to cost, organ availability, and associated risks[122] presenting challenges in determining the most suitable treatment for individual patients. Precision medicine emerges as a solution by analyzing an individual’s genetic makeup, lifestyle, and medical history to tailor treatment plans, addressing specific risk factors, and optimizing outcomes.[114]
Precision medicine for HF seeks to personalize treatment strategies based on individual characteristics, including genetic profiles, comorbidities, and responses to prior treatments. Utilizing cutting-edge molecular technologies such as genomics, proteomics, transcriptomics, metabolomics, and phenomics, combined with environmental and lifestyle factors, precision medicine identifies patient groups with similar disease profiles likely to benefit from targeted therapies.[123] This approach involves synthesizing extensive data collected through various methods to deeply characterize patient populations, with the primary goal of determining optimal care for individuals based on their unique profiles, moving away from reliance on population averages. The strength of precision medicine lies in its ability to synthesize rapidly evolving datasets, including information from clinical assessments, imaging, laboratory testing, next-generation sequencing (NGS), metabolomic and proteomic studies, as well as historical health record data.[124] Leveraging advanced systems biology and network analytical methods allows the discovery of new and unbiased relationships between health and disease factors within this expansive dataset.
In the context of HF, precision medicine holds three potential applications. First, it seeks to uncover the genetic basis of common HF causes such as CHD and CMP. Second, it aims to develop risk-stratification models for guiding counseling and therapeutic interventions, utilizing genetics to inform therapy choices based on predicted responses. Third, precision medicine focuses on identifying new targets for biological or pharmacological therapies, potentially repurposing existing medications or discovering new ones targeted at dysregulated pathways causing different forms of HF. This personalized approach allows for more precise and targeted disease management, potentially improving outcomes and enhancing the QoL for HF patients.
Recent advancements in precision medicine and advanced analytics have offered insights into the molecular pathways involved in HF, particularly distinguishing between patient groups with reduced and preserved ejection fraction. NGS has emerged as a powerful tool, uncovering novel gene targets across various medical fields and increasingly becoming financially feasible as a diagnostic modality. In contrast to genotyping panels, NGS provides the opportunity to discover not only novel mutations but also compound mutations that may collaborate to modify HF phenotypes and disease severity.[123] This technology holds the potential to revolutionize HF diagnosis and treatment, offering a more tailored and effective approach to managing this complex condition.
In addition to predictive and prognostic techniques, precision medicine can play a pivotal role in identifying potential responders to therapy before treatment initiation, particularly in the field of pharmacogenetics. Beta-blockers, extensively studied in pharmacogenomics for HF, exemplify this approach.[125] A notable example is the exploration of the Arg389Gly variant in the gene encoding the β1-adrenergic receptor (ADRB1). Pharmacological studies have revealed that receptors with the Arg389 variant exhibit greater basal and agonist-stimulated activity compared to those with the Gly389 variant. This difference in receptor activity has translated into significant mortality benefits for HF patients. Research has shown that patients homozygous for the ADRB1 Arg389 variant experienced a substantial 38% reduction in mortality when treated with bucindolol therapy, while Gly389 carriers did not derive a comparable benefit from the same treatment.[125,126] Studies have also shed light on RAAS-modifying drugs, specifically the variation in survival benefits associated with both endogenous ACE levels and the efficacy of ACE inhibition. These approaches have provided new insights into the role of genetic variation in predicting the severity of ventricular remodeling in CMP and CHD.[123]
The exploration of pharmacogenetics in HF illuminates the potential for a more personalized and effective therapeutic approach. By identifying genetic variants and their impact on the response to specific medications, health-care providers can make informed decisions about treatment plans that are more likely to benefit individual patients, enhancing the overall effectiveness of HF management and minimizing the risk of adverse reactions.
Precision medicine is poised to transform the future of CVD management due to the gradual onset and complex nature of these conditions. Despite its potential, the field is still evolving, with many necessary technologies in early stages. Limited research, hindered by ethical and social issues, along with considerations of cost-effectiveness and test availability, may impact the broader adoption of precision medicine in clinical practice.[127]
IMPORTANCE OF CONTINUED RESEARCH AND COLLABORATION
HF represents a significant challenge in CV medicine and surgery, leading to substantial cardiac fatalities and disabilities. Unfortunately, established therapies are underutilized due to factors such as limited access to care, poverty, and health-care system disarray.[128,129] To address CV risk factors and enhance HF treatment, continuous research and collaboration are essential.
Collaborative efforts offer a unique opportunity to pool resources, knowledge, and expertise from various institutions and countries. It is greatly needed to further explain the mechanisms of this illness and make the knowledge to benefit humanity. A multi-disciplinary approach to HF research fosters a holistic understanding of the condition, potentially leading to innovative treatments and interventions that can enhance patient outcomes. This collaboration can lead to the development of innovative treatments and interventions that can improve the outcomes for patients with HF. By working together, general practitioners, cardiologists, and other health-care providers can ensure that patients receive comprehensive and coordinated care. This multidisciplinary approach has been shown to significantly enhance patient care and improve outcomes for individuals with HF.
Continued research and collaboration play a pivotal role in addressing CV risk factors and advancing the field of HF management. By working together, professionals can better understand the intricate molecular pathways of HF, paving the way for personalized and effective treatments, along with improved prevention strategies. As the aging population and the prevalence of HF continue to rise, research and collaboration efforts are vital to address CV risk factors in the treatment of this challenging condition. It is essential for researchers, clinicians, and health-care organizations to collaborate and share findings to identify gaps in current approaches, develop new strategies, and ensure the best possible care for patients. By fostering collaboration and sharing knowledge and resources, health-care professionals can collectively create more effective prevention strategies to alleviate the burden of HF on health-care systems and society as a whole.
The significance of continued research and collaboration in addressing CV risk factors and treating HF cannot be overstated. These efforts hold the promise of improved diagnosis, treatment, and management of HF, ultimately benefiting patients worldwide.
CONCLUSION
The global burden of CVD poses a substantial public health challenge, significantly impacting both morbidity and mortality rates. The intricate connections between hypertension, DM, and HF underscore the complexity of CV health, emphasizing the urgent requirement for comprehensive prevention and management strategies.
As the prevalence of CVD continues to escalate, identifying innovative therapies becomes imperative. Recent research explores promising avenues, including SGLT-2 inhibitors, non-steroidal MRAs, and ERAs, offering hope for more targeted and effective treatments to address the pressing demand for solutions in preventing or delaying HF. Furthermore, this comprehensive review highlights the multifaceted landscape of CVD, emphasizing the need for a thorough understanding to enable effective prevention and management. Identifying individuals at risk and implementing proactive primary and secondary prevention strategies are pivotal. The focus on DM and hypertension is a critical risk factor for HF, underscores the importance of exploring innovative therapies to prevent or reverse its progression. While traditional interventions play a role, the need for novel and non-invasive approaches is evident.
DM significantly increases the risk of HF, involving elevated blood sugar levels, insulin resistance, glucose intolerance, and diabetic CMP. Innovative therapies, such as DPP-4 inhibitors, GLP-1 receptor agonists, and SGLT-2 inhibitors, exhibit varying impacts on HF outcomes, with SGLT-2 inhibitors emerging as promising therapeutic tools providing CV benefits beyond glycemic control. CGM and the development of a bionic pancreas signify significant strides in diabetes management, offering personalized and efficient approaches. Furthermore, combining SGLT-2 inhibitors with specific plant extracts presents innovative possibilities for restoring metabolic balance in cardiac tissue, potentially paving the way for comprehensive interventions addressing the complex interplay of diabetes and HF.
In summary, this review underscores the urgent need for innovative strategies to address the increasing prevalence of CVD, with a specific focus on preventing or delaying HF through the exploration of novel therapies targeting cardiac hypertrophy. Ongoing research in this field holds promise for improving patient outcomes and alleviating the societal burden imposed by CVDs.
Acknowledgments
The authors appreciate all members of Young Researcher forum of Nigeria, and Dr. Sunday Okafor (founder of Young Researcher forum of Nigeria) for his guidance.
Ethical approval
The Institutional Review Board approval is not required.
Declaration of patient consent
Patient’s consent was not required as there are no patients in this study.
Conflicts of interest
There are no conflicts of interest.
Use of artificial intelligence (AI)-assisted technology for manuscript preparation
The authors confirm that there was no use of artificial intelligence (AI)-assisted technology for assisting in the writing or editing of the manuscript and no images were manipulated using AI.
Financial support and sponsorship
None.
References
- Genetic architecture of human plasma lipidome and its link to cardiovascular disease. Nat Commun. 2019;10:4329. doi: 10.1038/s41467-019-11954-8
- [CrossRef] [PubMed] [Google Scholar]
- The epidemiology of cardiovascular disease in the UK 2014. Heart. 2015;101:1182-1189. doi: 10.1136/heartjnl-2015-307516
- [CrossRef] [PubMed] [Google Scholar]
- Vindoline-a natural product from Catharanthus roseus reduces hyperlipidemia and renal pathophysiology in experimental type 2 diabetes. Biomedicines. 2019;7:59. doi: 10.3390/biomedicines7030059
- [CrossRef] [PubMed] [Google Scholar]
- A prospective study of lipid profile and BMI as risk factors in MI in young [<40 yrs] in rural background. Acad J Med. 2019;2:51-53. doi: 10.21276/ajm.2019.2.2.14
- [CrossRef] [Google Scholar]
- Global burden of cardiovascular diseases and risk factors 1990-2019: Update from the GBD 2019 study. J Am Coll Cardiol. 2020;76:2982-3021. doi: 10.1016/j.jacc.2020.11.010
- [CrossRef] [PubMed] [Google Scholar]
- Incidence and prevalence of cardiovascular disease in English primary care: A cross-sectional and follow-up study of the Royal College of General Practitioners (RCGP) Research and Surveillance Centre (RSC) BMJ Open. 2018;8:e020282. doi: 10.1136/bmjopen-2017-020282
- [CrossRef] [PubMed] [Google Scholar]
- Hypertension and heart failure. Heart Fail Clin. 2020;16:99-106. doi: 10.1016/j.hfc.2019.09.001
- [CrossRef] [PubMed] [Google Scholar]
- Hypertension and heart failure. Heart Fail Clin. 2019;15:531-541. doi: 10.1016/j.hfc.2019.06.007
- [CrossRef] [PubMed] [Google Scholar]
- Heart failure in type 2 diabetes mellitus. Circ Res. 2019;124:121-141. doi: 10.1161/circresaha.118.311371
- [CrossRef] [PubMed] [Google Scholar]
- The pyruvate-lactate axis modulates cardiac hypertrophy and heart failure. Cell Metab. 2020;33:629-648.e10. doi: 10.1016/j.cmet.2020.12.003
- [CrossRef] [PubMed] [Google Scholar]
- Pathophysiology of cardiac hypertrophy and heart failure: Signaling pathways and novel therapeutic targets. Arch Toxicol. 2015;89:1401-1438. doi: 10.1007/s00204-015-1477-x
- [CrossRef] [PubMed] [Google Scholar]
- Pressure overload by suprarenal aortic constriction in mice leads to left ventricular hypertrophy without c-Kit expression in cardiomyocytes. Sci Rep. 2020;10:15318. doi: 10.1038/s41598-020-72273-3
- [CrossRef] [PubMed] [Google Scholar]
- Hypertensive heart disease In: StatPearls. Treasure Island, FL: StatPearls Publishing; 2023. Available from: https://www.ncbi.nlm.nih.gov/books/NBK539800 [Last accessed on 2024 Jan 10]
- [Google Scholar]
- Hypertension as a risk factor for heart failure. Curr Hypertens Rep. 2014;16:447. doi: 10.1007/s11906-014-0447-7
- [CrossRef] [PubMed] [Google Scholar]
- The transition from hypertension to heart failure: Contemporary update. JACC Heart Fail. 2017;5:543-551. doi: 10.1016/j.jchf.2017.04.012
- [CrossRef] [PubMed] [Google Scholar]
- Older adults, “malignant” left ventricular hypertrophy, and associated cardiac-specific biomarker phenotypes to identify the differential risk of new-onset reduced versus preserved ejection fraction heart failure. JACC Heart Fail. 2015;3:445-455. doi: 10.1016/j.jchf.2014.12.018
- [CrossRef] [PubMed] [Google Scholar]
- Arterial hypertension and chronic heart failure. Cor Et Vasa. 2013;55:e259-e263. doi: 10.1016/j.crvasa.2013.04.002
- [CrossRef] [Google Scholar]
- Pathways involved in the transition from hypertension to hypertrophy to heart failure. Treatment strategies. Heart Fail Rev. 2007;13:367-375. doi: 10.1007/s10741-007-9060-z
- [CrossRef] [PubMed] [Google Scholar]
- Importance of mean red cell distribution width in hypertensive patients. Cureus. 2016;8:e902. doi: 10.7759/cureus.902
- [CrossRef] [Google Scholar]
- Effect of endothelial microRNAs on blood pressure homeostasis. J Phys Fitness Sports Med. 2018;7:41-45. doi: 10.7600/jpfsm.7.41
- [CrossRef] [Google Scholar]
- Hypertension: Current trends and future perspectives. Br J Clin Pharmacol. 2021;87:3721-3736. doi: 10.1111/bcp.14825
- [CrossRef] [PubMed] [Google Scholar]
- Recent advances in managing primary hypertension. Fac Rev. 2020;9:4. doi: 10.12703/b/9-4
- [CrossRef] [PubMed] [Google Scholar]
- The potential role and rationale for treatment of heart failure with sodium-glucose co-transporter 2 inhibitors. Eur J Heart Fail. 2018;19:1390-1400. doi: 10.1002/ejhf.933
- [CrossRef] [PubMed] [Google Scholar]
- SGLT2 inhibitors and mechanisms of hypertension. Curr Cardiol Rep. 2018;20:1. doi: 10.1007/s11886-018-0943-5
- [CrossRef] [PubMed] [Google Scholar]
- Epidemiology, diagnosis and management of hypertension among patients on chronic dialysis. Nat Rev Nephrol. 2016;12(10):636-47. doi: 10.1038/nrneph.2016.129
- [CrossRef] [PubMed] [Google Scholar]
- New drug targets for hypertension: A literature review. Biochim Biophys Acta Mol Basis Dis. 2021;1867:166037. doi: 10.1016/j.bbadis.2020.166037
- [CrossRef] [PubMed] [Google Scholar]
- The non-steroidal mineralocorticoid receptor antagonist finerenone and heart failure with preserved ejection fraction. Cardiovasc Diabetol. 2023;22:162. doi: 10.1186/s12933-023-01899-0
- [CrossRef] [PubMed] [Google Scholar]
- Novel non-steroidal mineralocorticoid receptor antagonists in cardiorenal disease? Br J Pharmacol. 2022;179:3220-3234. doi: 10.1111/bph.15747
- [CrossRef] [PubMed] [Google Scholar]
- Erratum to: Non-steroidal mineralocorticoid receptor antagonists in cardiorenal disease. Eur Heart J. 2022;43:4391. doi: 10.1093/eurheartj/ehac425
- [CrossRef] [PubMed] [Google Scholar]
- Effect of finerenone on chronic kidney disease outcomes in type 2 diabetes. N Engl J Med. 2020;383:2219-2229. doi: 10.1056/nejmoa2025845
- [CrossRef] [PubMed] [Google Scholar]
- Novel therapeutics for the treatment of hypertension and its associated complications: Peptide-and nonpeptide-based strategies. Hypertens Res. 2021;44:740-755. doi: 10.1038/s41440-021-00643-z
- [CrossRef] [PubMed] [Google Scholar]
- Esaxerenone, a novel nonsteroidal mineralocorticoid receptor blocker (MRB) in hypertension and chronic kidney disease. J Hum Hypertens. 2021;35:148-156. doi: 10.1038/s41371-020-0377-6
- [CrossRef] [PubMed] [Google Scholar]
- Long-term phase 3 study of esaxerenone as mono or combination therapy with other antihypertensive drugs in patients with essential hypertension. Hypertens Res. 2019;42:1932-1941. doi: 10.1038/s41440-019-0314-7
- [CrossRef] [PubMed] [Google Scholar]
- Safety, tolerability, and pharmacokinetics of the mineralocorticoid receptor modulator AZD9977 in healthy men: A phase I multiple ascending dose study. Clin Transl Sci. 2019;13:275-283. doi: 10.1111/cts.12705
- [CrossRef] [PubMed] [Google Scholar]
- Effect of KBP-5074 on blood pressure in advanced chronic kidney disease: Results of the BLOCK-CKD study. Hypertension. 2021;78:74-81. doi: 10.1161/hypertensionaha.121.17073
- [CrossRef] [PubMed] [Google Scholar]
- An evaluation of KBP-5074 in advanced chronic kidney disease with uncontrolled hypertension. Expert Opin Investig Drugs. 2021;30:1017-1023. doi: 10.1080/13543784.2021.1985462
- [CrossRef] [PubMed] [Google Scholar]
- Neprilysin inhibition in heart failure: Mechanisms and substrates beyond modulating natriuretic peptides. Eur J Heart Fail. 2017;19:710-717. doi: 10.1002/ejhf.799
- [CrossRef] [PubMed] [Google Scholar]
- Neprilysin and natriuretic peptide regulation in heart failure. Curr Heart Fail Rep. 2016;13:151-157. doi: 10.1007/s11897-016-0292-x
- [CrossRef] [PubMed] [Google Scholar]
- Roles of natriuretic peptides and the significance of neprilysin in cardiovascular diseases. Biology. 2022;11:1017. doi: 10.3390/biology11071017
- [CrossRef] [PubMed] [Google Scholar]
- Targeted therapies in pulmonary arterial hypertension. Pharmacol Ther. 2014;141:172-191. doi: 10.1016/j.pharmthera.2013.10.002
- [CrossRef] [PubMed] [Google Scholar]
- Endothelin receptor antagonists for pulmonary arterial hypertension. Cochrane Database Syst Rev. 2021;3:CD004434. doi: 10.1002/14651858.cd004434.pub6
- [CrossRef] [PubMed] [Google Scholar]
- Effects of the dual endothelin receptor antagonist aprocitentan on body weight and fluid homeostasis in healthy subjects on a high sodium diet. Clin Pharmacol Ther. 2020;109:746-753. doi: 10.1002/cpt.2043
- [CrossRef] [PubMed] [Google Scholar]
- Targeted therapy of pulmonary arterial hypertension: Updated recommendations from the Cologne Consensus Conference 2018. Int J Cardiol. 2018;272:37-45. doi: 10.1016/j.ijcard.2018.08.082
- [CrossRef] [PubMed] [Google Scholar]
- Dual endothelin antagonist aprocitentan for resistant hypertension (PRECISION): A multicentre, blinded, randomised, parallel-group, phase 3 trial. Lancet. 2022;400:1927-1937. doi: 10.1016/s0140-6736(22)02034-7
- [CrossRef] [Google Scholar]
- Global estimates of diabetes prevalence for 2013 and projections for 2035. Diabetes Res Clin Pract. 2014;103:137-149. doi: 10.1016/j.diabres.2013.11.002
- [CrossRef] [PubMed] [Google Scholar]
- Diabetes mellitus and heart failure. Eur Cardiol. 2014;9:37-42. doi: 10.15420/ecr.2014.9.1.37
- [CrossRef] [Google Scholar]
- Role of diabetes in congestive heart failure: The Framingham study. Am J Cardiol. 1974;34:29-34. doi: 10.1016/0002-9149(74)90089-7
- [CrossRef] [PubMed] [Google Scholar]
- Gene polymorphisms and thyroid function in patients with heart failure. Endocrine. 2014;45:46-54. doi: 10.1007/s12020-013-9926-x
- [CrossRef] [PubMed] [Google Scholar]
- Prognostic value of blood glucose levels in diabetic patients upon admission and its outcomes in patients with acute coronary syndrome. Int J Surg Med. 2016;2:214-217. doi: 10.5455/ijsm.acs
- [CrossRef] [Google Scholar]
- Management of comorbid diabetes mellitus and worsening heart failure. JAMA. 2014;311:2379. doi: 10.1001/jama.2014.4115
- [CrossRef] [PubMed] [Google Scholar]
- Impaired glucose tolerance: A possible contributor to left ventricular hypertrophy and diastolic dysfunction. Int J Cardiol. 2007;118:76-80. doi: 10.1016/j.ijcard.2006.07.004
- [CrossRef] [PubMed] [Google Scholar]
- The impact of central obesity on the risk of hospitalization or death due to heart failure in type 1 diabetes: A 16-year cohort study. Cardiovasc Diabetol. 2021;20:153. doi: 10.1186/s12933-021-01340-4
- [CrossRef] [PubMed] [Google Scholar]
- Diabetes, hypertension, and cardiovascular disease: Clinical insights and vascular mechanisms. Can J Cardiol. 2018;34:575-584. doi: 10.1016/j.cjca.2017.12.005
- [CrossRef] [PubMed] [Google Scholar]
- The pathobiology of diabetic complications: A unifying mechanism. Diabetes. 2005;54:1615-1625. doi: 10.2337/diabetes.54.6.1615
- [CrossRef] [PubMed] [Google Scholar]
- Risk factors contributing to type 2 diabetes and recent advances in the treatment and prevention. Int J Med Sci. 2014;11:1185-1200. doi: 10.7150/ijms.10001
- [CrossRef] [PubMed] [Google Scholar]
- Heart failure, saxagliptin, and diabetes mellitus: Observations from the SAVOR-TIMI 53 randomized trial. Circulation. 2014;130:1579-1588. doi: 10.1161/circulationaha.114.010389
- [CrossRef] [PubMed] [Google Scholar]
- SGLT2 inhibitors in patients with heart failure with reduced ejection fraction: A meta-analysis of the EMPEROR-reduced and DAPA-HF trials. Lancet. 2020;396:819-829. doi: 10.1016/s0140-6736(20)31824-9
- [CrossRef] [PubMed] [Google Scholar]
- Effects of vildagliptin on ventricular function in patients with type 2 diabetes mellitus and heart failure. JACC Heart Fail. 2018;6:8-17. doi: 10.1016/j.jchf.2017.08.004
- [CrossRef] [PubMed] [Google Scholar]
- Heart failure in type 2 diabetes: Current perspectives on screening, diagnosis and management. Cardiovasc Diabetol. 2021;20:218. doi: 10.1186/s12933-021-01408-1
- [CrossRef] [PubMed] [Google Scholar]
- Glucagon-like peptide 1 receptor agonists and heart failure. Circulation. 2020;142:1205-1218. doi: 10.1161/circulationaha.120.045888
- [CrossRef] [PubMed] [Google Scholar]
- Anti-diabetic therapy and heart failure: Recent advances in clinical evidence and molecular mechanism. Life. 2023;13:1024. doi: 10.3390/life13041024
- [CrossRef] [PubMed] [Google Scholar]
- Effect of glucagon-like peptide-1 receptor agonists on prognosis of heart failure and cardiac function: A systematic review and meta-analysis of randomized controlled trials. Clin Ther. 2023;45:17-30. doi: 10.1016/j.clinthera.2022.12.006
- [CrossRef] [PubMed] [Google Scholar]
- Empagliflozin, cardiovascular outcomes, and mortality in type 2 diabetes. N Engl J Med. 2015;373:2117-2128. doi: 10.1056/nejmoa1504720
- [CrossRef] [PubMed] [Google Scholar]
- Empagliflozin increases cardiac energy production in diabetes. JACC Basic Transl Sci. 2018;3:575-587. doi: 10.1016/j.jacbts.2018.07.006
- [CrossRef] [PubMed] [Google Scholar]
- The failing heart relies on ketone bodies as a fuel. Circulation. 2016;133:698-705. doi: 10.1161/circulationaha.115.017355
- [CrossRef] [PubMed] [Google Scholar]
- SGLT2 inhibitors in patients with heart failure: A comprehensive meta-analysis of five randomised controlled trials. Lancet. 2022;400:757-767. doi: 10.1016/s0140-6736(22)01429-5
- [CrossRef] [PubMed] [Google Scholar]
- SGLT1: A potential drug target for cardiovascular disease. Drug Des Dev Ther. 2023;17:2011-2023. doi: 10.2147/dddt.s418321
- [CrossRef] [PubMed] [Google Scholar]
- Sodium-glucose co-transporter 2 inhibitors as an early, first-line therapy in patients with heart failure and reduced ejection fraction. Eur J Heart Fail. 2022;24:431-441. doi: 10.1002/ejhf.2397
- [CrossRef] [PubMed] [Google Scholar]
- DBI/ACBP is a targetable autophagy checkpoint involved in aging and cardiovascular disease. Autophagy. 2022;19:2166-2169. doi: 10.1080/15548627.2022.2160565
- [CrossRef] [PubMed] [Google Scholar]
- CARD9 as a potential target in cardiovascular disease. Drug Des Devel Ther. 2016;10:3799-3804. doi: 10.2147/dddt.s122508
- [CrossRef] [PubMed] [Google Scholar]
- Benefits and harms of intensive glycemic control in patients with type 2 diabetes. BMJ. 2019;367:l5887. doi: 10.1136/bmj.l5887
- [CrossRef] [PubMed] [Google Scholar]
- The effects of professional continuous glucose monitoring as an adjuvant educational tool for improving glycemic control in patients with type 2 diabetes. BMC Endocr Disord. 2021;21:79. doi: 10.1186/s12902-021-00742-5
- [CrossRef] [PubMed] [Google Scholar]
- Feasibility and acceptability of professional continuous glucose monitoring system in children with Type 1 diabetes mellitus: An observational study. J Diabetol. 2019;10:15. doi: 10.4103/jod.jod_13_18
- [CrossRef] [Google Scholar]
- Clinical use of continuous glucose monitoring in adults with type 2 diabetes. Diabetes Technol Ther. 2017;19:S4-S11. doi: 10.1089/dia.2017.0024
- [CrossRef] [PubMed] [Google Scholar]
- The effectiveness of continuous glucose monitoring in patients with type 2 diabetes: A systematic review of literature and meta-analysis. Diabetes Technol Ther. 2018;20:613-621. doi: 10.1089/dia.2018.0177
- [CrossRef] [PubMed] [Google Scholar]
- Multicenter, randomized trial of a bionic pancreas in type 1 diabetes. N Engl J Med. 2022;387:1161-1172. doi: 10.1056/nejmoa2205225
- [CrossRef] [PubMed] [Google Scholar]
- Addressing stem cell therapeutic approaches in pathobiology of diabetes and its complications. J Diabetes Res. 2018;2018:780643. doi: 10.1155/2018/780643
- [CrossRef] [PubMed] [Google Scholar]
- Stem cell therapy for heart failure. Methodist DeBakey Cardiovasc J. 2013;9:187. doi: 10.14797/mdcj-9-4-187
- [CrossRef] [PubMed] [Google Scholar]
- Efficacy of autologous bone marrow-derived stem cell transplantation in patients with type 2 diabetes mellitus. Stem Cells Dev. 2009;18:1407-1416. doi: 10.1089/scd.2009.0164
- [CrossRef] [PubMed] [Google Scholar]
- Endogenous reprogramming of alpha cells into beta cells, induced by viral gene therapy, reverses autoimmune diabetes. Cell Stem Cell. 2018;22:78-90.e4. doi: 10.1016/j.stem.2017.11.020
- [CrossRef] [PubMed] [Google Scholar]
- Plant extracts for type 2 diabetes : From traditional medicine to modern drug discovery. Antioxidants (Basel). 2021;10:81. doi: 10.3390/antiox10010081
- [CrossRef] [PubMed] [Google Scholar]
- From diabetes care to heart failure management: A potential therapeutic approach combining SGLT2 inhibitors and plant extracts. Nutrients. 2022;14:3737. doi: 10.3390/nu14183737
- [CrossRef] [PubMed] [Google Scholar]
- Mechanical circulatory support in the treatment of advanced heart failure. Am J Transplant. 2017;17:3020-3032. doi: 10.1111/ajt.14403
- [CrossRef] [PubMed] [Google Scholar]
- Mechanical cardiac assistance: Historical perspectives. Semin Thorac Cardiovasc Surg. 2000;12:207-219. doi: 10.1053/stcs.2000.18455
- [CrossRef] [PubMed] [Google Scholar]
- First reported use of the heartware HVAD in the US as bridge to transplant in an adolescent. Pediatr Transplant. 2012;16:E356-9. doi: 10.1111/j.1399-3046.2012.01718.x
- [CrossRef] [PubMed] [Google Scholar]
- Successful glucose lowering therapy triumphs in heart failure. EClinicalMedicine. 2021;37:100996. doi: 10.1016/j.eclinm.2021.100996
- [CrossRef] [PubMed] [Google Scholar]
- Pathophysiology and treatment of diabetic cardiomyopathy and heart failure in patients with diabetes mellitus. Int J Mol Sci. 2022;23:3587. doi: 10.3390/ijms23073587
- [CrossRef] [PubMed] [Google Scholar]
- Examining factors associated with excess mortality in older people (age ≥ 70 years) with diabetes-a 10-year cohort study of older people with and without diabetes. Diabet Med. 2016;34:387-395. doi: 10.1111/dme.13132
- [CrossRef] [PubMed] [Google Scholar]
- Diabetes, hypertension, and cardiovascular disease. Hypertension. 2001;37:1053-1059. doi: 10.1161/01.hyp.37.4.1053
- [CrossRef] [PubMed] [Google Scholar]
- Characterization of the potent, selective Nrf2 activator, 3-(Pyridin-3-Ylsulfonyl)-5-(Trifluoromethyl)-2H-Chromen-2-one, in cellular and in vivo models of pulmonary oxidative stress. J Pharmacol Exp Ther. 2017;363:114-125. doi: 10.1124/jpet.117.241794
- [CrossRef] [PubMed] [Google Scholar]
- The nuclear factor (erythroid-derived 2)-like 2 (Nrf2) activator dh404 protects against diabetes-induced endothelial dysfunction. Cardiovasc Diabetol. 2017;16:33. doi: 10.1186/s12933-017-0513-y
- [CrossRef] [PubMed] [Google Scholar]
- The antioxidant tempol attenuates pressure overload-induced cardiac hypertrophy and contractile dysfunction in mice fed a high-fructose diet. Am J Physiol Heart Circ Physiol. 2008;295:H2223-H2230. doi: 10.1152/ajpheart.00563.2008
- [CrossRef] [PubMed] [Google Scholar]
- Forsythiaside A inhibits hydrogen peroxide-induced inflammation, oxidative stress, and apoptosis of cardiomyocytes. Trop J Pharm Res. 2021;20:2029-2034. doi: 10.4314/tjpr.v20i10.3
- [CrossRef] [Google Scholar]
- Fixed combination of zofenopril plus hydrochlorothiazide in the management of hypertension: A review of available data. Vasc Health Risk Manag. 2006;2:341-349. doi: 10.2147/vhrm.2006.2.4.341
- [CrossRef] [PubMed] [Google Scholar]
- Beneficial effects of combined blockade of ACE and AT 1 receptor on intimal hyperplasia in balloon-injured rat artery. Arterioscler Thromb Vasc Biol. 2002;22:1299-1304. doi: 10.1161/01.atv.0000026298.00663.58
- [CrossRef] [PubMed] [Google Scholar]
- Quinapril. Cardiovasc Drug Rev. 1985;3:71-84. doi: 10.1111/j.1527-3466.1985.tb00472.x
- [CrossRef] [Google Scholar]
- Changes in mortality from heart failure-United States 1980-1995. JAMA. 1998;280:874. doi: 10.1001/jama.280.10.874
- [CrossRef] [PubMed] [Google Scholar]
- Sex differences in the application of evidence-based therapies for the treatment of acute myocardial infarction. Arch Intern Med. 2006;166:1164. doi: 10.1001/archinte.166.11.1164
- [CrossRef] [PubMed] [Google Scholar]
- Structured telephone support or non-invasive telemonitoring for patients with heart failure. Cochrane Database Syst Rev. 2015;2015:CD007228. doi: 10.1002/14651858.cd007228.pub3
- [CrossRef] [PubMed] [Google Scholar]
- Lifestyle modifications for preventing and treating heart failure. J Am Coll Cardiol. 2018;72:2391-2405. doi: 10.1016/j.jacc.2018.08.2160
- [CrossRef] [PubMed] [Google Scholar]
- 2013 ACCF/AHA guideline for the management of heart failure. J Am Coll Cardiol. 2013;62:e147-e239. doi: 10.1016/j.jacc.2013.05.019
- [CrossRef] [PubMed] [Google Scholar]
- A new multimodal geriatric discharge-planning intervention to prevent emergency visits and rehospitalizations of older adults: The optimization of medication in AGEd multicenter randomized controlled trial. J Am Geriatr Soc. 2011;59:2017-2028. doi: 10.1111/j.1532-5415.2011.03628.x
- [CrossRef] [PubMed] [Google Scholar]
- Current status and effect of outpatient cardiac rehabilitation after percutaneous coronary intervention in Japan. Circ Rep. 2021;3:122-130. doi: 10.1253/circrep.cr-20-0143
- [CrossRef] [PubMed] [Google Scholar]
- Palliative care needs of patients with advanced heart failure at a cardiac care unit in Mtrh, Kenya: A census study. Research Square [Preprint] 2023 doi: 10.21203/rs.3.rs-3153499/v1
- [CrossRef] [Google Scholar]
- Apps and online platforms for patients with heart failure. Card Fail Rev. 2020;6:e14. doi: 10.15420/cfr.2019.15
- [CrossRef] [PubMed] [Google Scholar]
- The present clinical treatment and future emerging interdisciplinary for heart failure: Where we are and what we can do. Intens Care Res. 2023;3:3-11. doi: 10.1007/s44231-023-00029-4
- [CrossRef] [Google Scholar]
- Cardiac-resynchronization therapy for mild-to-moderate heart failure. N Engl J Med. 2010;363:2385-95. doi: 10.1056/nejmoa1009540
- [CrossRef] [PubMed] [Google Scholar]
- Cutting-Edge innovations in cardiology: Exploring the latest advances in treating cardiovascular diseases. 2023. Available from: https://www.researchgate.net/publication/372532218_cutting-edge_innovations_in_cardiology_exploring_the_latest_advances_in_treating_cardiovascular_diseases_with_revolutionary_techniques_and_breakthrough_therapies [Last accessed on 2024 Jan 10]
- [Google Scholar]
- Wearable Devices to monitor and reduce the risk of cardiovascular disease: Evidence and opportunities. Ann Rev Med. 2021;72:459-471. doi: 10.1146/annurev-med-050919-031534
- [CrossRef] [PubMed] [Google Scholar]
- Nanotechnology in drug and gene delivery. Naunyn-Schmiedebergs Arch Pharmacol. 2022;395:769-787. doi: 10.1007/s00210-022-02245-z
- [CrossRef] [PubMed] [Google Scholar]
- SERCA2a gene therapy for heart failure: Ready for primetime? Mol Ther. 2008;16:1002-1004. doi: 10.1038/mt.2008.89
- [CrossRef] [PubMed] [Google Scholar]
- Gene therapy for ischaemic heart disease and heart failure. J Intern Med. 2021;290:567-582. doi: 10.1111/joim.13308
- [CrossRef] [PubMed] [Google Scholar]
- Gene therapy for heart failure. Korean Circ J. 2005;35:345. doi: 10.4070/kcj.2005.35.5.345
- [CrossRef] [Google Scholar]
- Potential future therapies for heart failure. Coron Artery Dis. 1999;10:401-406. doi: 10.1097/00019501-199909000-00009
- [CrossRef] [PubMed] [Google Scholar]
- Viral vectors for gene therapy and gene modification approaches. Biochem Eng J. 2016;108:98-115. doi: 10.1016/j.bej.2015.09.005
- [CrossRef] [Google Scholar]
- PD-L1 overexpression conveys tolerance of mesenchymal stem cell-derived cardiomyocyte?like cells in an allogeneic mouse model. J Cell Physiol. 2021;236:6328-6343. doi: 10.1002/jcp.30299
- [CrossRef] [PubMed] [Google Scholar]
- Meeting the need for regenerative therapies I: Target-based incidence and its relationship to U.S. spending, productivity, and innovation. Tissue Eng Part B Rev. 2012;18:139-154. doi: 10.1089/ten.teb.2011.0454
- [CrossRef] [PubMed] [Google Scholar]
- Stem cell therapy in heart failure. Highl Sci Eng Technol. 2023;36:1222-1229. doi: 10.54097/hset.v36i.6206
- [CrossRef] [Google Scholar]
- Stem cell therapy in patients with heart failure. Methodist DeBakey Cardiovascu J. 2013;9:6.
- [CrossRef] [PubMed] [Google Scholar]
- Pre-conditioned mesenchymal stem cells: A better way for cell-based therapy. Stem Cell Res Ther. 2013;4:63. doi: 10.1186/scrt213
- [CrossRef] [PubMed] [Google Scholar]
- Stem cell therapy for heart failure: The science and current progress. Fut Cardiol. 2008;4:285-298. doi: 10.2217/14796678.4.3.285
- [CrossRef] [PubMed] [Google Scholar]
- The discrete-continuous, global optimisation of an axial flow blood pump. Flow Turbul Combust. 2019;104:777-793. doi: 10.1007/s10494-019-00100-5
- [CrossRef] [Google Scholar]
- Perspective on precision medicine in paediatric heart failure. Clin Sci. 2017;131:439-448. doi: 10.1042/cs20160414
- [CrossRef] [PubMed] [Google Scholar]
- Emerging role of precision medicine in cardiovascular disease. Circ Res. 2018;122:1302-1315. doi: 10.1161/circresaha.117.310782
- [CrossRef] [PubMed] [Google Scholar]
- Heart failure in the era of precision medicine: A scientific statement from the American Heart Association. Circ Genom Precis Med. 2019;12:458-485. doi: 10.1161/hcg.0000000000000058
- [CrossRef] [PubMed] [Google Scholar]
- Towards precision medicine in heart failure. Nat Rev Cardiol. 2021;18:745-762. doi: 10.1038/s41569-021-00566-9
- [CrossRef] [PubMed] [Google Scholar]
- Precision medicine and the future of cardiovascular diseases: A clinically oriented comprehensive review. J Clin Med. 2023;12:1799. doi: 10.3390/jcm120517909
- [CrossRef] [PubMed] [Google Scholar]
- Unstable angina: Is it time for a requiem? Circulation. 2013;127:2452-2457. doi: 10.1161/CIRCULATIONAHA.113.001258
- [CrossRef] [PubMed] [Google Scholar]
- Forecasting the impact of heart failure in the United States. Circ Heart Fail. 2013;6:606-619. doi: 10.1161/hhf.0b013e318291329a
- [CrossRef] [PubMed] [Google Scholar]