Translate this page into:
Mupirocin/hydroxyapatite composite suspended in transcutol P-spiked hydrogel: In vitro characterization and in vivo wound healing assessment
*Corresponding author: Ibilola Mary Cardoso-Daodu, PhD Department of Pharmaceutics and Pharmaceutical Technology, Faculty of Pharmacy, University of Lagos, College of Medicine, Lagos, Nigeria. icardoso@unilag.edu.ng
-
Received: ,
Accepted: ,
How to cite this article: Ojo OE, Ilomuanya MO, Cardoso-Daodu IM, et al. Mupirocin/hydroxyapatite composite suspended in transcutol P-spiked hydrogel: In vitro characterization and in vivo wound healing assessment. Am J Pharmacother Pharm Sci 2024:11.
Abstract
Objectives:
Natural hydroxyapatite (HAP) has been utilized as a drug carrier owing to its excellent bioactivity and biocompatibility. This study is aimed at formulating mupirocin/hydroxyapatite composite suspended in hydrogel. The appropriate quantity of the penetration enhancer (Transcutol-P®) was also investigated.
Materials and Methods:
The HAP was isolated from bovine bone by hydrothermal treatment, calcined at 1000oC and held for 2 hours in an electric furnace to remove the organic contents. The bones were milled, sifted using 150 µm mesh sieve and characterized. Olive oil, which contains oleic acid, was utilized as a natural capping agent to prevent agglomeration of the particles in the formulation. The quantity of Transcutol-P® was varied with mupirocin used as the active pharmaceutical ingredient for the management of acute wound in Wistar rats. In this animal study, the wound closure rate was evaluated.
Results:
The formulation with the 0.6%v/v, of Transcutol-P® gave the best wound closure rate of 30.05 mm2/day. The in-vitro study showed that the formulation containing 0.6%v/v Transcutol-P® released 63.9% of the drug after 75 minutes while 42.4% was released at the same time interval when the concentration of the penetration enhancer was increased to 1.2%v/v. The mupirocin-encapsulated HAP hydrogel composite showed high resistance against staphylococcus saprophyticus with inhibition zone of 37.3 mm.
Conclusion:
The mupirocin encapsulated in HAP allows for sustained release of the antibiotic and thus serves as a veritable drug carrier suitable for wound healing applications. Transcutol-P® (0.6%v/v) is effective in facilitating drug release, which is reflected in the increased wound closure rate in Wistar rats.
Keywords
Hydrogel
Staphylococcus saprophyticus
Wound healing
Mupirocin
Oleic acid
INTRODUCTION
Hydroxyapatite (HAP) is a naturally occurring form of calcium phosphate and the main mineral constituent of bones and teeth. Natural HAP has physical and chemical characteristics similar to that of bone which makes it biocompatible. Owing to its other outstanding properties, namely bioactivity, non-toxicity, and non-inflammatory nature, it is broadly used in biomedical applications.[1,2] Natural HAP can be biogenically isolated from mammalian bone (goat, bovine, horse, and camel), shell sources (seashell and eggshell), marine sources (fish bone and scale), and mineral sources (limestone).[3] It can also be prepared synthetically by a combination of chemical precursors such as calcium nitrate tetrahydrate, ammonium dihydrogen phosphate, calcium hydroxide, and ortho-phosphoric acid among others.[4] It has been ascertained in different research works that HAP extracted from biogenic resources exhibits more superior biological properties over the one synthesized from chemicals.[5] The natural HAP is majorly obtained from bones by expelling the organic phase (collagen) and water present in the bone leaving behind HAP through the calcination process.
HAP applications in the biomedical domain cannot be overemphasized. These range from bone substitutes, and implants, to drug delivery systems.[6] In this study, HAP isolated from bovine bones (BHAP) was used as a drug carrier for acute wound management. Wounds are defined as the breakage or disruption of the skin caused by trauma or physiological conditions. Wounds have a profound negative effect on the health-care systems and economy worldwide.[7] It was recently reported that almost one billion people suffer from acute and chronic wounds globally with people of 65 years and above accounting for 85% of this population.[8] The United States of America spends an average of 50 billion US dollars annually on chronic wounds management.[9] Wound healing is a normal biological process in the human body. It is achieved through four precise and highly programmed phases, namely hemostasis, inflammation, proliferation, and the remodeling phase. For a wound to heal successfully, all four phases must occur in the proper sequence and time frame. A myriad of factors can interfere with any of the four phases in wound healing, thus causing improper or impaired wound healing.[10] It is generally known that the technique of delivering antimicrobials using particles with high surface area offers several advantages that can increase antimicrobial activity as the drugs are protected from enzymatic destruction, released in a controlled manner, and delivered selectively to the target tissues. This, in turn, accelerates wound healing.[11]
This study is aimed at formulating mupirocin (MUP) encapsulated BHAP suspended in hydrogel and investigating its wound healing ability while varying the quantity penetration enhancer (Transcutol P®) used in the formulations. Transcutol-P® is a potent, non-toxic, and biodegradable, solvent that significantly enhances percutaneous penetration of drugs. The hydrogel formulation composition may include a polymer, active pharmaceutical ingredient (API), humectant, preservatives, and penetration enhancers. Hydrogel-forming polymers are classified as natural polymers such as proteins (collagen, gelatin, xanthan, and gellam gum), polysaccharides (agar, alginic acid, and guar gum), synthetic polymers such as carbomer (carbopol-940, carbopol-934, and carbopol-941), polyacrylamide, polyvinyl alcohol, polyethylene and its copolymers, and inorganic substances such as aluminum hydroxide or bentonite.[12]
MUP, used as an API, is a topical antibiotic isolated from the bacterium, Pseudomonas fluorescens. It is produced as a mixture of four pseudomonic acids (A, B, C, and D) which is used to treat superficial infections mainly caused by Gram-positive bacteria, and it acts by inhibition of protein synthesis in targeted bacteria.[13] Recently, polymer hydrogels have become widely attractive as dermal drug delivery agents because they result in faster drug release to the targeted site, regardless of the hydrophilicity or lipophilicity of its load.[14] It can be easily washed off from the skin and it also provides good stability.[12] At present, and to the best of our knowledge, there is no hydrogel formulation loaded with an arsenal of a drug delivery agent, antibiotic, and permeation enhancer for enhancing wound healing. The MUP/HAP composite suspended in hydrogel along with Transcutol-P® in one formulation, as a bioactive wound dressing will efficiently and competently provide a therapeutic solution to the prognosis of acute wound healing.
MATERIALS AND METHODS
Materials
Bovine femur bones from approximately 36-month-old animals utilized in this study were obtained at Magboro main market in Ogun State, Nigeria. The materials used include MUP powder as API (CAS number 12650-69-0 Shaanxi Top Pharmaceutical Chemical Co., Limited, Shaanxi, China), Mueller–Hilton Agar (HiMedia Laboratory Pvt., Limited, India), Carbopol 940 (Hubei New Desheng Materials Technology Co., Limited, Ezhou city, Hubei province, China), Transcutol-P® (Gattefosse Sas Saint-Priest Cedex-France), olive oil used as the source of oleic acid (Goya En Espana, S.A.U. Sevilla, Spain), acetic acid (AA) (99.8% purity Sigma-Aldrich, Germany), methyl paraben, propyl paraben (VA-SUDHA Chemicals PVT LTD, India). Other chemical reagents and solvents were of analytical grade and used in this study without further purification.
Bone preparation and calcination
The adhering tissues present in the bone samples were removed manually before defatting. After drying, they were charged into a laboratory electric furnace (Carbonite, England) with a 16,000 cm3 heating chamber for calcination at 1000°C for 2 h and allowed to cool to ambient temperature in the furnace before discharging them. This temperature is within the range proven by Manalu et al. as being suitable for the isolation of pure HAP.[15,16] The calcined bones were hammer milled to facilitate the bone size reduction to <10 mm using Broyeur Clero® (model 13634, 1.5 kV, France).
Then later subjected to ball milling, employing a Faure® balling machine (model 28A20:92, France). The particles were shifted with a 150 µm mesh sieve before characterizing the isolated BHAP.[15]
Wound healing formulation
Capping of BHAP particles
BHAP (10 g) was poured into a beaker with 100 mL of distilled water, also 3 mL of olive oil (OLF) (as an oleic acid source) was added. The solution was stirred for an hour with a magnetic stirrer at 1000 rpm. It was filtered and the residue was stirred 3 times in ethanol to remove the OLF. The solution was again filtered and the capped BHAP (residue) was dried in an oven at 75°C for 24 h and pulverized using laboratory porcelain mortar and pestle.
Drug loading of BHAP particles
MUP powder (4 g) was poured into a beaker containing 100 mL of ethanol. Then, 10 g of capped BHAP was dispersed into the beakers containing MUP-ethanol solution and stirred with a magnetic stirrer at 1000 rpm for 15 min. The suspension was centrifuged at 4000 rpm for 5 min and a small portion of the supernatant was taken for ultraviolet-visible spectroscopy (UV-VIS) analysis. The solution was dried in the oven at 37°C until the ethanol was fully evaporated.
UV-infrared spectroscopy analysis
The UV-VIS analysis was carried out on the supernatant obtained after centrifugation to establish the final concentration of the MUP present in the ethanol, which was used to determine the encapsulation efficiency (EE) and drug loading capacity (DLC) of the BHAP.
Hydrogel formulations
Carbopol-940 (500 mg) was poured into four beakers each containing 50 mL of distilled water according to the number of formulations to be prepared. They were stirred, covered, and left for an hour to swell. Triethanolamine was added in drops to adjust the pH to ~5.5 and increase the viscosity. The hydrogel pH was determined in triplicate. A minute quantity of propyl paraben (0.01 g) and methylparaben (0.02 g) were added as preservatives; 20 mL of propylene glycol was added as a humectant for wound hydration. Transcutol-P® was also added to each of them in different volumes as shown in Table 1. They were thoroughly stirred for homogeneity.
Formulation | MUP (% w/v) |
BHAP (% w/v) |
Transcutol (% v/v) |
Carbopol (% w/v) | Propyl paraben (% w/v) |
Methyl paraben (% w/v) | Propylene glycol (% v/v) |
pH |
---|---|---|---|---|---|---|---|---|
F1 | - | 10 | 1.2 | 0.5 | 0.01 | 0.02 | 20 | 5.58 |
F2 | 2 | 10 | 0.6 | 0.5 | 0.01 | 0.02 | 20 | 5.73 |
F3 | 2 | 10 | 1.2 | 0.5 | 0.01 | 0.02 | 20 | 5.95 |
F4 | 2 | 10 | 1.8 | 0.5 | 0.01 | 0.02 | 20 | 6.10 |
MUP: Mupirocin, BHAP: HAP isolated from bovine bones, HAP: Hydroxyapatite.
Formulation of MUP-BHAP hydrogel composite
MUP (5 g) loaded-capped BHAP (MUP: BHAP) was added to the obtained hydrogel to prepare 2% w/v of MUP in the hydrogel formulations, which is the standard concentration of MUP in the conventional topical dosage form. It was made up to 100 mL with distilled water and thoroughly stirred with a spatula for homogeneity. The formulations’ compositions and pH are shown in Table 1.
Morphological and chemical characterizations
Scanning electron microscopy (SEM)
The particle shape and morphology were determined using an ASPEX 3020 model variable pressure SEM operated with an electron intensity beam of 15 kV and equipped with a Noran–Voyager energy dispersive spectroscope. The samples to be observed under the SEM were mounted on a conductive carbon imprint left by the adhesive tape prepared by placing the samples on the circular holder and coated for 5 min to enable it to conduct electricity. For energy dispersive spectroscope analysis, samples were analyzed at an accelerating voltage of 15 kV.
Fourier-transform infrared (FTIR) spectroscopy
The functional groups present in the BHAP and MUP: BHAP were confirmed using FTIR, Agilent Technologies® (USA) FTIR analyzer in the range of 4000–650 cm−1. Dehydration of the samples by vacuum drying at 45°C was carried out and later positioned over the diamond crystal for the FTIR analysis. Twenty scans were recorded for each spectrum and where necessary, smoothing was done to diminish the noise without any peak loss.
X-ray diffractometry (XRD)
Crystallographic analysis of the samples was performed on an EMPYERN XRD-600 diffractometer (45kV, 40 mA) with a CuKα (λ = 1.54). Radiation over the scanning range 5° to 80° was utilized.
Determination of EE and DLC
The UV-VIS analysis was carried out to reveal the unencapsulated MUP present in the ethanol after drug loading. This was input into equations 1 and 2 to determine the EE and DLC of the BHAP particles, respectively.[16]
Where:
EE is encapsulation efficiency, DLC is drug loading capacity, TDL is total drug loaded, UED is an unencapsulated drug, TED is a total encapsulated drug, and TPW is the total BHAP particle weight.
Biological characterizations
In vitro drug release study
The release study of MUP in MUP: BHAP/hydrogel scaffolds was done using a modified in situ glass vial system, and a 2.5 mL part of each of the formulations was immersed into 50 mL of phosphate buffer solution (pH 7.4). The samples were incubated at 37 ± 0.5°C and stirred at 100 rpm using a magnetic stirrer. Aliquots of each sample (1 mL) were taken from the release medium at specific intervals (0, 5, 15, 30, 60, and 75 min) and replaced with a fresh buffer solution of the same quantity to maintain sink condition. The solution was subsequently filtered using a Millipore filter of 0.45 um porosity. The resultant solution was analyzed by high-performance liquid chromatography.[17]
Evaluation of antibacterial activities
The antimicrobial activities of MUP, MUP: BHAP, and BHAP were estimated against pathogenic Gram-positive bacteria such as Staphylococcus saprophyticus and Klebsiella pneumonia and Gram-negative such as Staphylococcus aureus and Escherichia coli. Exactly 38 g of Mueller–Hinton Agar (as recommended) was dissolved in 1000 mL of distilled water, poured into a bottle, and autoclaved for 45 min to sterilize and fully dissolve the agar in the distilled water. Once the temperature reached room temperature, 20 mL was poured into each of the four sterile petri dishes (one for each bacterium) in the laminar flow cupboard to avoid any form of contamination. After solidification, the bacteria were introduced to the agar in the Petri dishes with the aid of sterile swab sticks beside a flaming burner to avoid contamination. A marker was used to divide the Petri dish for each sample, and 6-mm diameter paper discs were put at each division. Normal saline solution was used to dissolve the MUP whereas AA was used to dissolve MUP: BHAP and BHAP. Ten microliter was taken from the solution of each sample and introduced in drops to each of the paper discs. They were incubated for 24 h; the zones of inhibition were measured in triplicate with a metric ruler.[18,19]
In vivo wound healing study
Twenty-five male rats 16 weeks old were procured from Priceless Pet Animals, Badagry, Lagos, Nigeria, with an average weight of 181 g. They were housed at room temperature of 28 ± 2°C and relative humidity of 75 ± 10% in a well-ventilated environment and adequately fed. Each of the rats was kept in a semi-transparent polymer cage of dimension 50 × 36 × 23 cm with a 23 × 34 cm netted opening on top. They were allowed to acclimatize for 10 days before the start of the experiment. All the guidelines approved by the University of Lagos College of Medicine’s Health Research Ethical Committee (CMUL/HREC/03/21/829) were followed during the experiments. The wound incisions were made on the dorsum (back of the body) of the rats. After cleaning the wounds with methylated spirit, the length and width of the wounds were measured with a veneer caliper to calculate the wound area. The hydrogel formulations F1, F2, F3, and F4 were applied for 22 days at 2-day intervals and then dressed in gauze and plaster. The control animal wounds were only cleaned and dressed; no formulation was applied.[20]
Histological studies
After the 21-day post-treatment of the wound, the rats were humanely euthanized, and the incised wound area of each rat was sampled by trimming to include the dermis and the hypodermis. Each of the trimmed samples was kept in sample bottles filled with 10% neutral buffered formalin to preserve the tissues. After paraffin embedding, 3–4 µm sections were prepared. Representative sections were then stained with hematoxylin and eosin. On individual rat skin sections, a light microscopic examination for histological profiles was performed.[20]
Statistical analysis
The experiments were carried out in triplicate and reported as mean ± standard deviation. Statistical difference between the mean values was determined by one-way analysis of variance (ANOVA) or two-way ANOVA followed by Tukey or Bonferroni post hoc test, respectively (if applicable) using the GraphPad® 6 Prism software (GraphPad Software, La Jolla, CA).
RESULTS
Bone calcination
HAP was extracted from the bovine bones by means of the thermal decomposition process at 1000°C, the weight of the bone charged into the furnace was reduced to 315 g after the process from 500 g, which was a 37% weight reduction.
EE and DLC
The UV-VIS analysis revealed that out of 4 g (TDL) of MUP loaded for encapsulation, 0.13 g (UED) was found in the ethanol. This implies that only 3.87 g was actually encapsulated (TED) by the capped BHAP. EE, which is the percentage of drug that is successfully entrapped into the particles was 96.75%. Furthermore, DLC which is the amount of drug encapsulated by 1 g of BHAP was calculated as 387 mg/g. EE was close to the value obtained (98.67%) by Weerasuriya et al. when HAP was loaded with copper bis (8-hydroxyquinoline) but the DLC of 2.9522 mg/g was less than what was obtained (387mg/g) in this study.[21,22]
Morphological and chemical characteristics
SEM
The SEM results of BHAP particles before and after capping are shown in Figure 1. There are no distinctive differences in their morphology. Contrarily, as indicated by Energy dispersive x-ray spectroscopy (EDX), there was a difference in the major elemental compositions before and after BHAP capping as shown in Table 2. There was a reduction in the weight percentage of calcium and phosphorus after capping from 66.19 wt% to 60.10 wt% and 23.08 wt% to 10.33 wt%, respectively. There was a slight increase in carbon and sodium content. Furthermore, oxygen was not present in the calcined BHAP but was present in capped BHAP (20.22 wt%).[22]
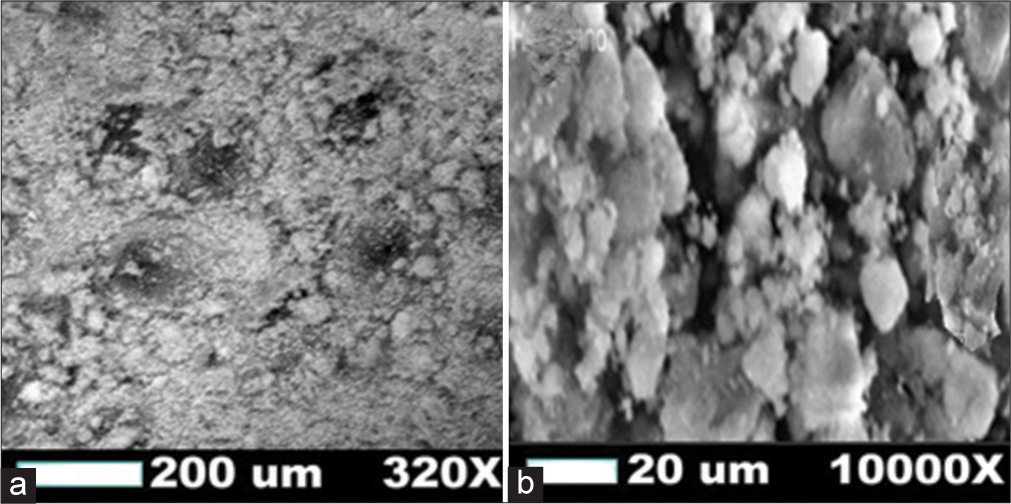
- The micrograph of BHAP (a) before and (b) after capping. BHAP: HAP isolated from bovine bones, HAP: Hydroxyapatite
Elements | BHAP% | Capped BHAP% |
---|---|---|
Calcium | 66.19 | 60.10 |
Phosphorus | 23.08 | 10.33 |
Oxygen | 20.22 | |
Carbon | 1.10 | 3.8 |
Sodium | 0.75 | 1.25 |
Others | 8.88 | 4.3 |
BHAP: HAP isolated from bovine bones, HAP: Hydroxyapatite.
FTIR spectroscopy
The FTIR spectra of the BHAP and MUP: BHAP in the region 4000–650 cm−1 are shown in Figure 2. FTIR spectra were comparatively analyzed, and this was done to confirm the active presence of MUP in the drug-loaded BHAP. MUP and oleic acid bands were identified. Band shifts in the MUP: BHAP spectrum were noticed. The main peak of phosphate (PO43−) corresponding to asymmetric stretching vibrations (V3) in the BHAP spectrum was at a wavelength of 1021 cm−1 and 7.2% transmittance, but in MUP: BHAP, it appeared at a wavelength of 1017 cm−1 and 17.99% transmittance, there was a slight shift of the peak and change in intensity after drug loading. It was also observed that the CO3 band at 1416 cm−1 with 90.8% transmittance in BHAP came to 78% in MUP: BHAP. There was no band shift observed in hydroxyl (OH-), which remained at 3571 cm−1 but there was a change in peak intensity. All the characteristic peaks of MUP were observed in MUP: BHAP in FTIR spectrum which according to Li et al., were 1712, 2859, 2929, 1150, and 1052 cm−1. Furthermore, some of the peaks in MUP: BHAP spectrum corresponded with what Ibarra-Hurtado et al. pointed out as the characteristic bands of oleic acid at 2923, 2854, 1708, 1464, 1285, and 936 cm−1. The intensities of these bands are strongly influenced by the composition and molar absorptivity of each characteristic vibration.[22-25]
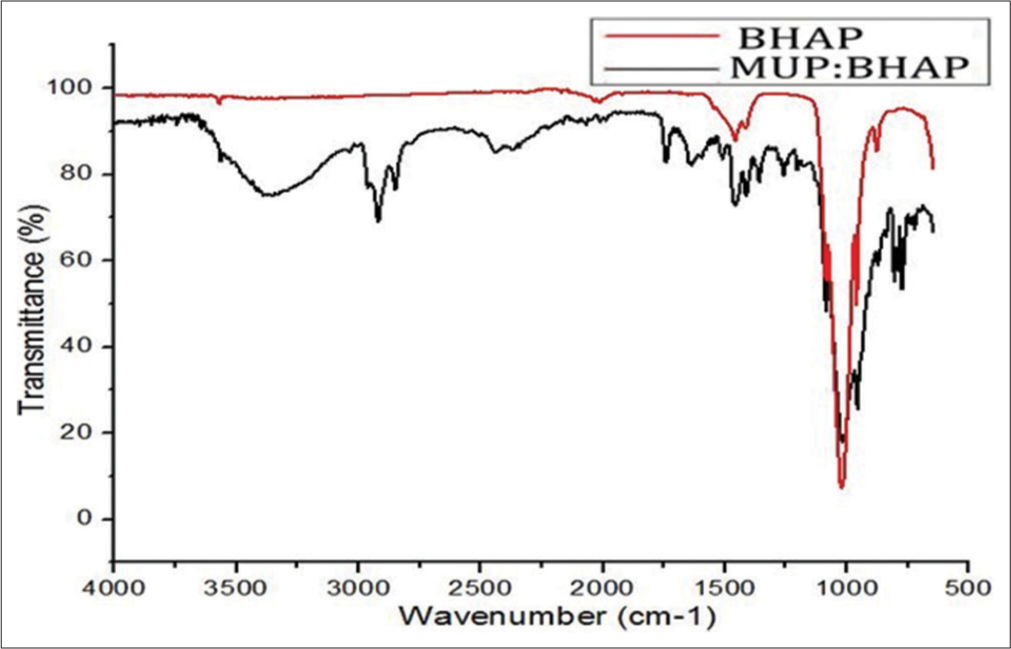
- Fourier-transform infrared spectra of BHAP and MUP: BHAP. MUP: Mupirocin, BHAP: HAP isolated from bovine bones, HAP: Hydroxyapatite
XRD
The phase composition analysis was performed on BHAP and MUP: BHAP samples. The comparison between their XRD patterns is shown in Figure 3. Some peaks were not revealed in XRD patterns of BHAP but appeared in MUP: BHAP patterns. The high-intensity patterns which are shown as MUP peaks in Figure 3 appeared at 2θ = ~ (6, 18, 19.5, and 24°). The intensity of the characteristic peak of HAP at 32° in BHAP (2871) reduced to 1730 in MUP: BHAP after drug loading.
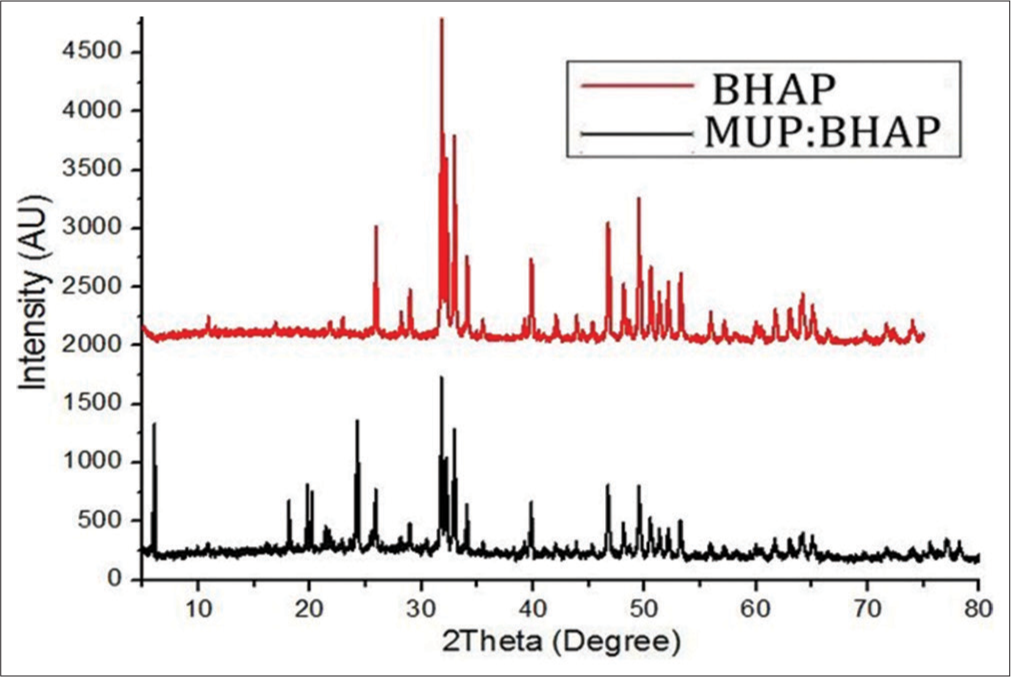
- X-ray diffractometry of BHAP and MUP: BHAP. MUP: Mupirocin, BHAP: HAP isolated from bovine bones. HAP: Hydroxyapatite.
Biological characteristics
In vitro drug release study
The ability of the formulation to effectively release MUP is important to the effectiveness of the formulations. BHAP must have the ability to release API at the site of action. The drug release of MUP was obtained at R2 = 0.999 at a wavelength of 230 nm. The graph of the percentage of MUP released from BHAP in each of the formulations against time in minutes is shown in Figure 4. The percentage of drug released at 75 min in formulations F2, F4, and F3 was 63.90%, 61.33%, and 42.40%, respectively, whereas for F1, there was no drug release.[24]
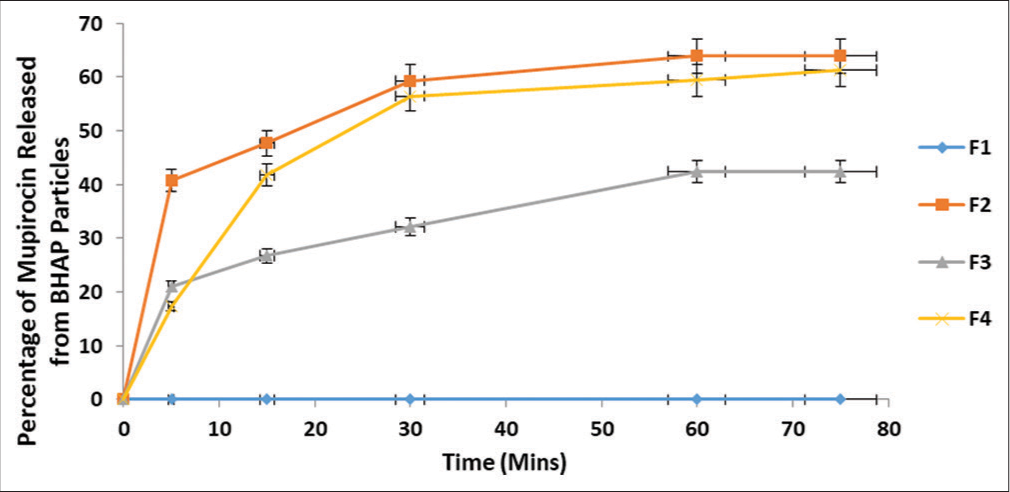
- Percentage of MUP release from BHAP within a specified period of time. Results are expressed as mean ± standard deviation (n = 3). For all data sets (P < 0.05). MUP: Mupirocin, BHAP: HAP isolated from bovine bones. HAP: Hydroxyapatite, F: Formulation.
In vivo wound healing study and histopathology
In this animal study, the wound closure rate (WCR) was used for evaluation. The control animals showed the lowest WCR of 14.45 mm2/day, while the animals treated with F1, F2, F3, and F4 had 15.94, 30.05, 28.65, and 20.99 mm2/day, respectively. The animals treated with F1, despite having no API showed a relatively better healing rate than the control animals. F2 that contained 0.6% v/v Transcutol-P® gave the highest WCR (30.05 mm2/day). This is closely followed by F3 (28.65 mm2/day) with 1.2% v/v of the penetrant and F4 (20.99 mm2/day) with 1.8% v/v. The graphical and pictorial representations of wound closure area in the treated animals and control are shown in Figures 5 and 6, respectively. It was observed that most of the animals that had high WCR experienced weight gain whereas the ones with wound expansion or lower closure rate showed weight loss [Figure 5]. The histology of the control animal with no treatment showed fibrocollagenous tissue infiltrated by chronic inflammatory cells. There were also thin-walled blood vessels within the stroma. Animals treated with F1 showed stratified keratinizing squamous epithelium with skin adnexal structures within the dermis but there was minimal inflammation with no structural abnormality in the cell (atypia). In animals treated with F2, there was no atypia seen but minimal inflammation was present while the one treated with F3 showed skin tissue with infiltration of acute and chronic inflammatory cells within the dermis. The overlying epidermis was ulcerated. Animals treated with F4 showed an abnormal epidermis. The dermis was infiltrated by chronic inflammatory cells, some of which were clusters.
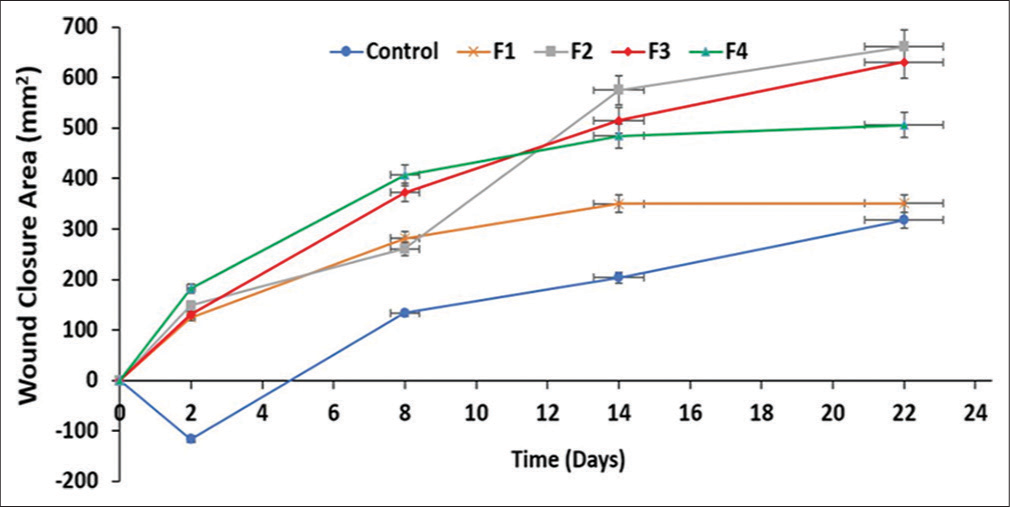
- Graphical representation of wound closure area in the animals treated with the formulations and control. Results are expressed as mean ± standard deviation (n = 3). For all data sets (P < 0.05). F: Formulation.
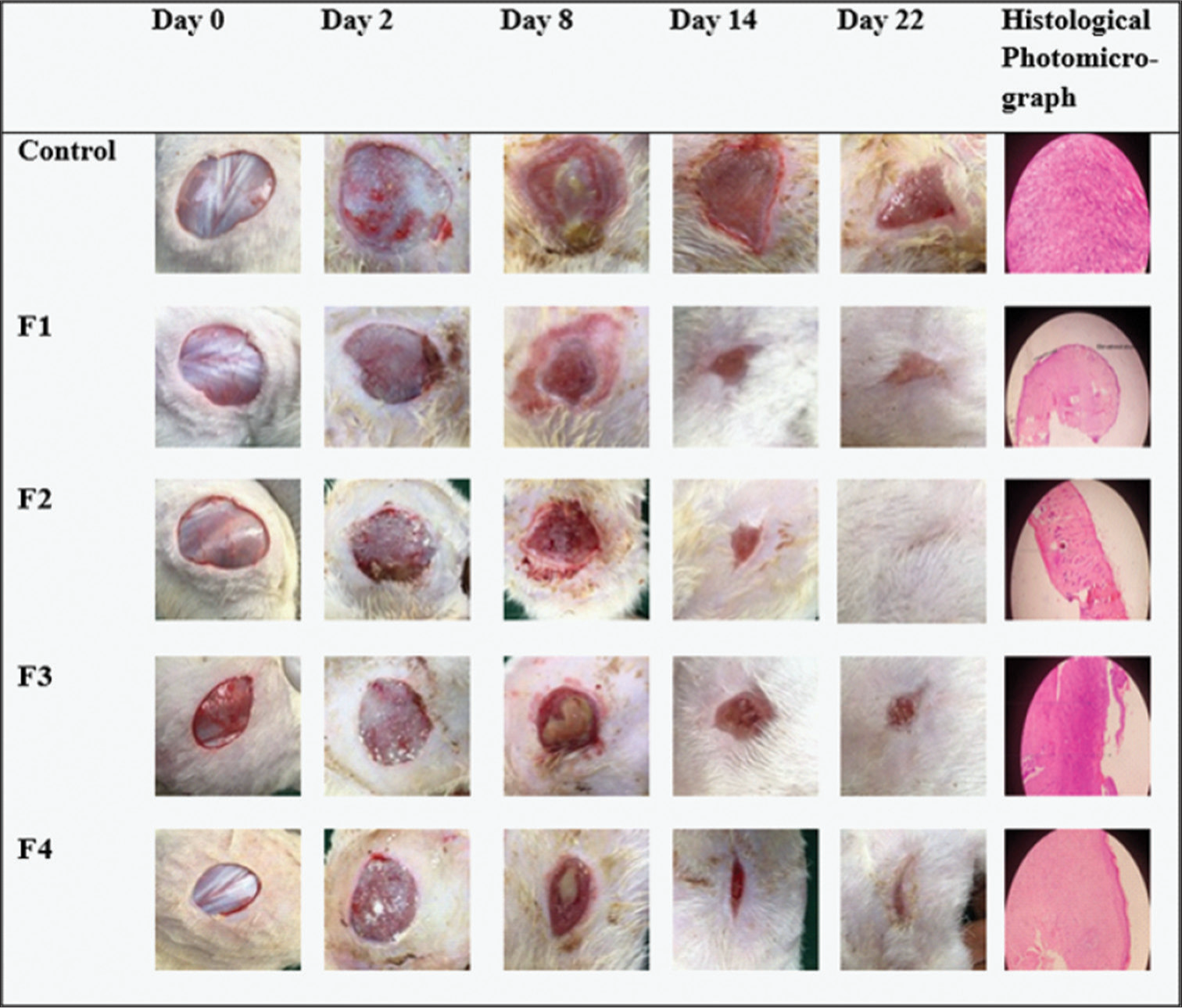
- Representative images of wounds showing contraction as the treatment period elapsed and histological photomicrograph. F: Formulation.
Antibacterial activities of MUP and BHAP
The diameter of the zone of inhibition, which indicates resistance of the bacteria growth, is shown in Figure 7a-d. The BHAP did not show any inhibition, zone against any of the bacteria used in this study. The average diameter of the inhibition zone for MUP against the S. saprophyticus, S. aureus, K. pneumonia, and E. coli was 4.25, 3.37, 1.37, and 1.17 cm, respectively. Mupirocin is an effective antibacterial drug, often used as a topical ointment to treat several varieties of topical wounds.[19] It showed an excellent activity against S. saprophyticus and S. aureus as revealed in Figure 7e. This implies that the API has a high level of antimicrobial activity against S. saprophyticus more than any other bacterium used in this study, while E. coli showed the highest level of resistance to the API with a 1.17 cm inhibition zone. The zone of inhibition of MUP: BHAP measured against the antibacterial activities of S. saprophyticus, S. aureus, K. pneumonia, and E. coli was 3.73, 2.88, 0.85, and 0.85 cm, respectively.
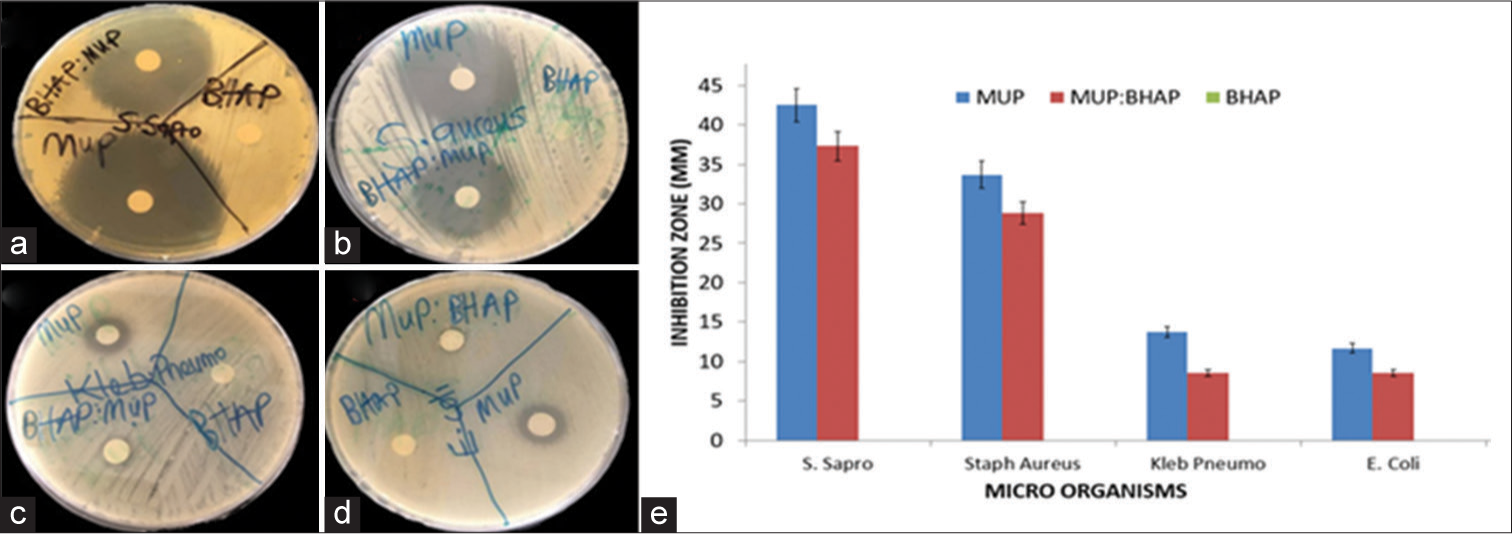
- Evaluation of antibacterial activity of MUP, MUP: BHAP, and BHAP on (a) Staphylococcus saprophyticus, (b) Staphylococcus aureus, (c) Klebsiella pneumonia, and (d) Escherichia coli (e) Inhibition zone of varying organisms when exposed to MUP, MUP: BHAP and BHAP. MUP: Mupirocin, BHAP: HAP isolated from bovine bones, HAP: Hydroxyapatite.
DISCUSSION
Naturally derived HAP has been demonstrated to be more suitable than the costly and synthetic HAP with a low degree of bioactivity which can be excellently substituted by cheap and natural HAP for biomedical applications. Transcutol-P is a potent non-toxic biodegradable solubilizer that significantly enhances percutaneous penetration of drugs. Transcutol has a superior solubilization power, unique physicochemical properties, and a well-established safety profile which makes it an ideal permeation enhancer. Transcutol-P in this study was used in different concentrations to readily penetrate the stratum corneum and interact with water of the intercellular path to modify skin permeation of the MUP.[25] In the calcination process at 1000°C, the 37% weight loss represents the mass of organic matter (collagen) dispelled from the bone. Therefore, increase in weight loss leads to more collagen being eliminated. The pH of the F1, F2, F3, and F4 hydrogel as shown in Table 1 is 5.58, 5.73, 5.95, and 6.10, respectively. The pH was made close to normal human skin pH (4.0–6.3) because an acidic wound bed is an additional benefit that can hasten up the wound toward healing.[26] The pH changes modulate biological and biochemical processes in wound healing.[27] Wound pH reduces as the different stages of healing are reached. Therefore, pH is a useful tool for predicting the likelihood of successful healing of a wound. The micrographs of the BHAP before and after capping revealed that the process did not alter the morphology of the BHAP.[28] Oxygen was not present in BHAP before capping but present after, it might have been introduced by the capping agent. This was equally responsible for the reduction in calcium and phosphorus weight percentage in capped BHAP. The XRD and FTIR analysis of the MUP: BHAP showed the characteristic bands and peaks of MUP which means that it was still potentially active in BHAP after drug loading.[29] These characteristic peaks of MUP in the XRD were intense and sharp indicating its crystalline nature. The changes in the intensities and the position of the corresponding absorption bands in FTIR spectra may be due to the change in the molecular environment of BHAP which might have been caused by MUP.[28,30]
The formulations were designed for the sustained release of MUP as the active ingredient into the wound bed and to facilitate the healing process. The in vitro study revealed that F2 had the highest percentage of drug release (63.90%) whereas F3 had the lowest (42.4%) and F4 had 61.3% after 75 min. F1 was expected to have a zero release rate since it did not contain any API. F2 having the highest drug release rate was shown to be the best of all the formulations as revealed during in vivo wound healing study. This may be attributed to it having the least quantity of Transcutol-P® (0.6% v/v). The control animal having the lowest WCR of 14.45 mm2/day was expected as there were no formulations administered. Similarly, the animal treated with F1, despite having no API in the formulation still showed a better healing response than the control as the hydrogel applied could aid the epithelization and skin tissue reconstruction on full thickness wound.[29]
F2 containing the least quantity of the penetrant gave the highest WCR because it gave the best support to increased wound blood flow; percentage occupied by collagen in granulation tissue and increase in re-epithelialization rate which led to quicker closure of the wound.[29] It could be deduced that at lower volumes of the penetrant WCR is faster. There was a strong connection between the WCR and the weight of the animals used in the study. The animals with the highest WCR in each of the formulation groups experienced weight gain whereas the ones with the lowest rates of wound expansion suffered weight loss. This is possible because an open wound can create a complex catabolic process that can cause systemic deficiencies, while this catabolic state can result in nutritional compromise that can lead to weight loss.[31] The fibro collagenous tissue of the control animal was infiltrated by chronic inflammatory cells because no formulation was applied to promote the healing which led to unhindered multiplication of the bacteria. In animals treated with F2, histology showed skin tissue with denuded epidermis, which might be caused by friction generated when applying the formulation or when removing the dressing. The dermis of animals treated with F4 which was infiltrated by chronic inflammatory cells might be a result of the slow closure rate of its wound. The pure MUP sample showed excellent activity against S. saprophyticus and S. aureus which are the major bacteria found in infected wounds but comparatively showed less resistance against K. pneumonia and E. coli as indicated in Figure 6. There was no inhibition zone observed around BHAP against any of the bacteria used in this study because it does not possess any antimicrobial activity on its own. However, the inhibition zones of MUP: BHAP disc were large but not as large as those around the MUP disc. This could be a result of the different drug release rates between the MUP and the MUP: BHAP. Furthermore, BHAP having no inhibition zone might have slightly reduced the activity of the MUP against the bacteria. The limitation of this study lies in the fact that the effect of Transcutol-P as a penetration enhancer on other natural forms of HAP such as HAP sourced from bones of marine animals or animal teeth was not investigated or compared to the bovine-sourced HAP used in this study.
CONCLUSION
This study is aimed at formulating MUP -HAP composite suspended in hydrogel and determining the appropriate volume of the penetration enhancer (Transcutol-P®) for the formulation in the management of acute wounds. This study confirmed that the quantity of Transcutol-P® present in the wound healing formulation can significantly influence its WCR. Formulation F2 with 0.6% v/v of Transcutol-P® had the highest drug release of 63.90% of MUP in 75 min during in vitro drug release study, hence gave the best WCR of 30.05 mm2/day. MUP-HAP composite suspended in the hydrogel with penetration enhancer (Transcutol-P®) can serve as a prototype for further development of a wound dressing formulation that enhances rapid and healthy wound healing in acute wounds.
Ethical approval
The research/study was approved by the Institutional Review Board at the University of Lagos College of Medicine’s Health Research Ethical Committee, number CMUL/HREC/03/21/829, dated March 2021.
Declaration of patient consent
Patient’s consent is not required as there are no patients in this study.
Conflicts of interest
There are no conflicts of interest.
Use of artificial intelligence (AI)-assisted technology for manuscript preparation
The authors confirm that there was no use of artificial intelligence (AI)-assisted technology for assisting in the writing or editing of the manuscript and no images were manipulated using AI.
Financial support and sponsorship
None.
References
- Hydroxyapatite: Preparation, and its biomedical applications. Adv Chem Eng Sci. 2018;8:225-240. doi: 10.4236/aces.2018.84016
- [CrossRef] [Google Scholar]
- Nano-hydroxyapatite and its contemporary applications. J Dent Res Sci Dev. 2014;1:15-37. doi: 10.4103/2348-3407.126135
- [CrossRef] [Google Scholar]
- Syntheses of hydroxyapatite from natural sources. Heliyon. 2019;5:e01588. doi: 10.1w016/j.heliyon.2019.e01588
- [CrossRef] [PubMed] [Google Scholar]
- Synthesis and characterization of hydroxyapatite nanoparticle. Malays J Anal Sci. 2017;21:136-148. doi: 10.17576/mjas-2101-16
- [CrossRef] [Google Scholar]
- Study of bovine hydroxyapatite obtained by calcination at low heating rates and cooled in furnace air. J Mater Sci. 2016;51:4431-4441. doi: 10.1007/s10853-016-9755-4
- [CrossRef] [Google Scholar]
- Synthesis of hydroxyapatite for biomedical applications. Adv Colloid Interfac. 2017;249:321-330. doi: 10.1016/j.cis.2017.04.007
- [CrossRef] [PubMed] [Google Scholar]
- Nano-drug delivery systems in wound treatment and skin regeneration. J Nanobiotechnology. 2019;17:82. doi: 10.1186/s12951-019-0514-y
- [CrossRef] [PubMed] [Google Scholar]
- Wound healing: Time to look for intelligent, “natural” immunological approaches? BMC Immunol. 2017;18:39-46. doi: 10.1186/s12865-017-0207-y
- [CrossRef] [PubMed] [Google Scholar]
- Wound healing: A cellular perspective. Physiol Rev. 2019;99:665-706. doi: 10.1152/physrev.00067.2017
- [CrossRef] [PubMed] [Google Scholar]
- Factors affecting wound healing. J Dent Res. 2020;89:219-229. doi: 10.1177/0022034509359125
- [CrossRef] [PubMed] [Google Scholar]
- Biofilm penetration, triggered release and in vivo activity of inhaled liposomal amikacin in chronic Pseudomonas aeruginosa lung infections. J Antimicrob. 2008;61:859-868. doi: 10.1093/jac/dkn059
- [CrossRef] [PubMed] [Google Scholar]
- Status of fatty acids as skin penetration enhancers-a review. Curr Drug Deliv. 2009;6:274-279. doi: 10.2174/156720109788680877
- [CrossRef] [PubMed] [Google Scholar]
- Topical gels as drug delivery systems: A review. Int J Pharm Sci Rev Res. 2013;23:374-382.
- [Google Scholar]
- Mupirocin: Applications and production. Biotechnol Lett. 2019;41:495-502. doi: 10.1007/s10529-019-02670-w
- [CrossRef] [PubMed] [Google Scholar]
- Formulation and evaluation of wound healing activity of polyherbal gel. Int J Pharm Biol Sci. 2017;7:158-167. doi: ijpbs_59f3f32115ae7
- [Google Scholar]
- Characterization of hydroxyapatite derived from bovine bone. Asian J Appl Sci. 2015;3:758-765.
- [Google Scholar]
- Optimization of the entrapment efficiency and release of ambroxol hydrochloride alginate beads. J Appl Pharm Sci. 2015;1:13-19. doi: 10.7324/japs.2015.50403
- [CrossRef] [Google Scholar]
- Polylactic acid-based electrospun fiber and hyaluronic acid-valsartan hydrogel scaffold for chronic wound healing. Beni-Suef Univ. J. Basic Appl. Sci. 2020;9:31-40. doi: 10.1186/s43088-020-00057-9
- [CrossRef] [Google Scholar]
- Evaluation of antibacterial activity of some medicinal plants extracts commonly used in Algerian traditional medicine against some pathogenic bacteria. Pharmacogn J. 2018;10:507-512. doi: 10.5530/pj.2018.3.83
- [CrossRef] [Google Scholar]
- Development of curcumin-loaded liposomes in lysine-collagen hydrogel for surgical wound healing. Beni-Suef Univ J Basic Appl Sci. 2022;11:100. doi: 10.1186/s43088-022-00284-2
- [CrossRef] [Google Scholar]
- Development of electrospun keratin/coenzyme Q10/poly vinyl alcohol nanofibrous scaffold containing mupirocin as potential dressing for infected wounds. Future J Pharm Sci. 2020;6:25. doi: 10.1186/s43094-020-00043-z
- [CrossRef] [Google Scholar]
- Encapsulation of anticancer drug copper bis(8-hydroxyquinoline) in hydroxyapatite for pH-sensitive targeted delivery and slow release. Mater Sci Eng. 2017;71:206-213. doi: 10.1016/j.msec.2016.10.010
- [CrossRef] [PubMed] [Google Scholar]
- Electrospun PCL/mupirocin and chitosan/lidocaine hydrochloride multifunctional double layer nanofibrous scaffolds for wound dressing applications. Int J Nanomed. 2018;13:5287-5299. doi: 10.2147/ijn.s177256
- [CrossRef] [PubMed] [Google Scholar]
- Development of Emulgel delivery of mupirocin for treatment of skin infection. Recent Pat Antiinfect Drug Discov. 2020;15:137-156. doi: 10.2174/1386207323999200819153404
- [CrossRef] [PubMed] [Google Scholar]
- Synthesis and characterization of magnetite/PLGA/chitosan nanoparticles. Mater Res Express. 2015;2:095010. doi: 10.1088/2053-1591/2/9/095010
- [CrossRef] [Google Scholar]
- Skin penetration and permeation properties of Transcutol®-neat or diluted mixtures. AAPS Pharm SciTech. 2018;19:3512-3533. doi: 10.1208/s12249-018-1196-8
- [CrossRef] [PubMed] [Google Scholar]
- Influence of acidic pH on wound healing in vivo: A novel perspective for wound treatment. Int J Mol Sci. 2022;23:13655. doi: 10.3390/ijms232113655
- [CrossRef] [PubMed] [Google Scholar]
- The effect of pH on the extracellular matrix and biofilms. Adv Wound Care. 2015;4:431-439. doi: 10.1089/wound.2014.0538
- [CrossRef] [PubMed] [Google Scholar]
- Characterisation of natural hydroxyapatite extracted from bovine cortical bone ash. J Ceram. 2009;10:129-138.
- [Google Scholar]
- Silver Sulphadiazine-xanthan gum-hyaluronic acid composite hydrogel for wound healing: Formulation development and in vivo evaluation. Niger J Pharm Res. 2020;16:21-29. doi: 10.4314/njpr.v16i1.3
- [CrossRef] [Google Scholar]
- Accelerated healing of diabetic wounds by NorLeu(3)-angiotensin (1-7) Expert Opin Investig Drugs. 2011;20:1575-1581. doi: 10.1517/13543784.2011.61997
- [CrossRef] [PubMed] [Google Scholar]